Little Brown Myotis, Northern Myotis and Tri-colored Bat recovery strategy
Read the recovery strategy for the Little Brown Myotis, Northern Myotis and Tri-colored Bat, three mammal species at risk in Ontario.
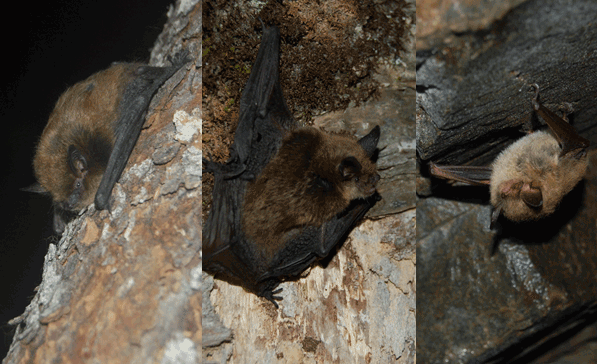
Cover illustration: Photos by Jordi Segers
About the Ontario recovery strategy series
This series presents the collection of recovery strategies that are prepared or adopted as advice to the Province of Ontario on the recommended approach to recover species at risk. The Province ensures the preparation of recovery strategies to meet its commitments to recover species at risk under the Endangered Species Act, 2007 (ESA) and the Accord for the Protection of Species at Risk in Canada.
What is recovery?
Recovery of species at risk is the process by which the decline of an endangered, threatened, or extirpated species is arrested or reversed, and threats are removed or reduced to improve the likelihood of a species’ persistence in the wild.
What is a recovery strategy?
Under the ESA a recovery strategy provides the best available scientific knowledge on what is required to achieve recovery of a species. A recovery strategy outlines the habitat needs and the threats to the survival and recovery of the species. It also makes recommendations on the objectives for protection and recovery, the approaches to achieve those objectives, and the area that should be considered in the development of a habitat regulation. Sections 11 to 15 of the ESA outline the required content and timelines for developing recovery strategies published in this series.
Recovery strategies are required to be prepared for endangered and threatened species within one or two years respectively of the species being added to the Species at Risk in Ontario list. Recovery strategies are required to be prepared for extirpated species only if reintroduction is considered feasible.
What’s next?
Nine months after the completion of a recovery strategy a government response statement will be published which summarizes the actions that the Government of Ontario intends to take in response to the strategy. The implementation of recovery strategies depends on the continued cooperation and actions of government agencies, individuals, communities, land users, and conservationists.
For more information
To learn more about species at risk recovery in Ontario, please visit the Ministry of the Environment, Conservation and Parks Species at Risk webpage.
Recommended citation
Humphrey, Christy and Heather Fotherby. 2019. Recovery Strategy for the Little Brown Myotis (Myotis lucifugus), Northern Myotis (Myotis septentrionalis) and Tri-colored Bat (Perimyotis subflavus) in Ontario. Ontario Recovery Strategy Series. Prepared by the Ministry of the Environment, Conservation and Parks, Peterborough, Ontario. vii + 35 pp. + Appendix. Adoption of the Recovery Strategy for the Little Brown Myotis (Myotis lucifugus), the Northern Myotis (Myotis septentrionalis), and the Tri-colored Bat (Perimyotis subflavus) in Canada (Environment and Climate Change Canada 2018).
Cover illustration: Photos by Jordi Segers
ISBN (HTML) 978-1-4868-3940-7
ISBN (PDF) 978-1-4868-3941-4
Content (excluding illustrations) may be used without permission with appropriate credit to the source, except where use of an image or other item is prohibited in the content use statement of the adopted federal recovery strategy.
Cette publication hautement spécialisée « Recovery strategies prepared under the Endangered Species Act, 2007 », n’est disponible qu’en anglais en vertu du Règlement 411/97 qui en exempte l’application de la Loi sur les services en français. Pour obtenir de l’aide en français, veuillez communiquer avec recovery.planning@ontario.ca.
Acknowledgments
The authors would like to thank the GIS department at NRSI for contributing the maps and Jordi Segers (Canadian Wildlife Health Co-operative) for contributing the photographs.
In addition, we would like to thank all of those who provided technical assistance. This includes the following individuals who provided information on the distribution of these species in Ontario: Derek Morningstar (Myotistar, LGL Limited), Kristin Jonasson, Yolanda Morbey and Chris Guglielmo (University of Western Ontario), Christina Davy, Lesley Hale and Mark Browning (Ministry of Natural Resources and Forestry), Judith Girard (Environment and Climate Change Canada), Lauren Hooton (Trent University), Bridget Schulte-Hostedde and Ed Morris (Ontario Parks), Toby Thorne and Eryk Matczak (Toronto Zoo), Lauren Trute (Ministry of the Environment, Conservation and Parks), Cathy Entwistle and Josh Vandermeulen. The following individuals provided additional information on these species, both in Ontario and generally: Jay Fitzsimmons, Anne Marie Laurence and Michelle Karam (Ministry of Natural Resources and Forestry). We would also like to thank those who provided information to help inform home ranges for these species, including Carl Herzog (New York State Department of Environmental Conservation) and Dave Yates (Biodiversity Research Institute).
Declaration
The recovery strategy for the Little Brown Myotis (Myotis lucifugus), Northern Myotis (Myotis septentrionalis) and Tri-colored Bat (Perimyotis subflavus) was developed in accordance with the requirements of the Endangered Species Act, 2007 (ESA). This recovery strategy has been prepared as advice to the Government of Ontario, other responsible jurisdictions and the many different constituencies that may be involved in recovering the species.
The recovery strategy does not necessarily represent the views of all individuals who provided advice or contributed to its preparation, or the official positions of the organizations with which the individuals are associated.
The recommended goals, objectives and recovery approaches identified in the strategy are based on the best available knowledge and are subject to revision as new information becomes available. Implementation of this strategy is subject to appropriations, priorities and budgetary constraints of the participating jurisdictions and organizations.
Success in the recovery of this species depends on the commitment and cooperation of many different constituencies that will be involved in implementing the directions set out in this strategy.
Responsible jurisdictions
Ministry of Environment, Conservation and Parks
Environment and Climate Change Canada – Canadian Wildlife Service, Ontario
Parks Canada Agency
Executive summary
The Endangered Species Act, 2007(ESA) requires the Minister of the Environment, Conservation and Parks (MECP) to ensure recovery strategies are prepared for all species listed as endangered or threatened on the Species at Risk in Ontario (SARO) List. Under the ESA, a recovery strategy may incorporate all or part of an existing plan that relates to the species.
The Little Brown Myotis (Myotis lucifugus), Northern Myotis (Myotis septentrionalis) and Tri-colored Bat (Perimyotis subflavus) are listed as endangered on the SARO List. These species are listed as endangered under the federal Species at Risk Act(SARA). Environment and Climate Change Canada (ECCC) published the Recovery Strategy for the Little Brown Myotis (Myotis lucifugus), the Northern Myotis (Myotis septentrionalis), and the Tri-colored Bat (Perimyotis subflavus) in Canada in 2018 to meet its requirements under the SARA. This recovery strategy is hereby adopted under the ESA. With the additions indicated below, the enclosed strategy meets all of the content requirements outlined in the ESA.
The Critical Habitat section of the federal recovery strategy provides an identification of some of the critical habitat for these species (as defined under the SARA). Critical habitat was not fully identified in the federal recovery strategy due to a lack of information. Identification of critical habitat is not a component of a recovery strategy prepared under the ESA. However, it is recommended that the approach used to identify the critical habitat that was identified in the federal recovery strategy, along with information in this addendum and any new scientific information pertaining to the Little Brown Myotis, Northern Myotis and Tri-colored Bat, and the areas they occupy, be considered when developing a habitat regulation under the ESA.
When developing the habitat regulation recommendation, detailed information available on roosting Little Brown Myotis, Northern Myotis and Tri-colored Bat during the maternity and swarming/hibernation seasons (where available), along with detailed information available on home range sizes and foraging habitat for these species, was considered. This included data from Ontario as well as from other jurisdictions. As such, it is recommended that hibernacula, swarming sites and maternity sites be prescribed as regulated habitat for Little Brown Myotis, Northern Myotis, and Tri-colored Bat. In addition, it is recommended that the regulated habitat for all hibernacula and swarming sites include areas of associated foraging and roosting resources, and that regulated maternity habitat for each species include the maternity roost site as well as areas of associated foraging resources.
Foraging habitat: Foraging habitat is important for multiple life stages and processes and is therefore included within habitat recommendations for these components (i.e., maternity roosts, hibernacula and swarming sites).
Hibernacula and swarming sites: It is recommended that all known hibernacula and swarming sites for Little Brown Myotis, Northern Myotis and/or Tri-colored Bat be prescribed as habitat in a habitat regulation, with the exception of any sites which are no longer suitable (e.g., structure collapsed or destroyed, access blocked to prevent bats from entering, permanently flooded) and any anthropogenic structures resided in by humans. It is further recommended that foraging and roosting resources within 2,600 m of a hibernaculum and/or swarming site be identified as habitat in the habitat regulation. The area should extend 2,600 m from all known or suspected entrances of a hibernaculum, or total underground extent of a hibernaculum, if known, and/or the concentrated area of swarming activity.
Maternity sites: For Little Brown Myotis, Northern Myotis and Tri-colored Bat, it is recommended that maternity habitat be identified based on the contiguous ecosite or contiguous anthropogenic site where all known observations of roosting adult females and juveniles between May 15 and July 31 have been made, unless the habitat is no longer suitable, or bats are no longer roosting at the site. Maternity sites may also be identified based on any feature where two or more Little Brown Myotis, Northern Myotis or Tri-colored Bat have been observed in, or observed to exit from, the feature, between May 15 and July 31. It is further recommended that foraging areas within 2,400 m of the boundary of a maternity site for Little Brown Myotis, 450 m of the boundary of a maternity site for Northern Myotis, and 920 m of the boundary of a maternity site for Tri-colored Bat be identified as supporting foraging habitat in the habitat regulation, with the combined maternity roosting and foraging habitat not exceeding 1,800 ha, 63 ha, and 265 ha in total, respectively.
Future research on the roosting, migratory, foraging and maternity habitats used by these species, as recommended in the federal recovery strategy, should be used to re-assess the suitability of the area recommended for inclusion in habitat regulations for these species, and to update the habitat regulations for these species as needed.
1.0 Adoption of federal recovery strategy
The Endangered Species Act, 2007(ESA) requires the Minister of the Environment, Conservation and Parks to ensure recovery strategies are prepared for all species listed as endangered or threatened on the Species at Risk in Ontario (SARO) List. Under the ESA, a recovery strategy may incorporate all or part of an existing plan that relates to the species.
The Little Brown Myotis (Myotis lucifugus), Northern Myotis (Myotis septentrionalis) and Tri-colored Bat (Perimyotis subflavus) are listed as endangered on the SARO List. These species are listed as endangered under the federal Species at Risk Act (SARA). Environment and Climate Change Canada (ECCC) prepared the Recovery Strategy for the Little Brown Myotis (Myotis lucifugus), the Northern Myotis (Myotis septentrionalis), and the Tri-colored Bat (Perimyotis subflavus) in Canada in 2018 to meet its requirements under the SARA. This recovery strategy is hereby adopted under the ESA. With the additions indicated below, the enclosed strategy meets all of the content requirements outlined in the ESA.
1.1 Species assessment and classification
The following lists are assessment and classification information for the Little Brown Myotis (Myotis lucifugus), Northern Myotis (Myotis septentrionalis) and Tri-colored Bat (Perimyotis subflavus). Note: The glossary provides definitions for the abbreviations and technical terms in this document.
Little Brown Myotis:
- SARO List Classification: Endangered
- SARO List History: Endangered (2013)
- COSEWIC Assessment History: Endangered (2012), Endangered (2013)
- SARA Schedule 1: Endangered
- Conservation Status Rankings: G-rank: G3; N-rank: N3; S-rank: S4
Northern Myotis:
- SARO List Classification: Endangered
- SARO List History: Endangered (2013)
- COSEWIC Assessment History: Endangered (2012), Endangered (2013)
- SARA Schedule 1: Endangered
- Conservation Status Rankings: G-rank: G1G2; N-rank: N2N3; S-rank: S3
Tri-colored Bat:
- SARO List Classification: Endangered
- SARO List History: Endangered (2016)
- COSEWIC Assessment History: Endangered (2012), Endangered (2013)
- SARA Schedule 1: Endangered
- Conservation Status Rankings: G-rank: G2G3; N-rank: N2N3; S-rank: S3?
1.2 Distribution, abundance and population trends
Sections 3.2 and 7.1 of the federal recovery strategy (Appendix 1) provide a description of the populations, distribution and critical habitat of Little Brown Myotis, Northern Myotis and Tri-colored Bat in Ontario. Since the publication of the federal recovery strategy, additional observations of these species have been reported and additional data have been considered in the preparation of this addendum.
Distribution of hibernacula and swarming sites
A review of data available from multiple sources and recent communications has identified several hibernacula and swarming sites in addition to what was included in mapping of critical habitat within the federal recovery strategy. Figure 1 and Figure 2 depict the general locations of all hibernacula and swarming sites that have been identified to date in southern and northern Ontario, respectively. Mapping provided in the federal recovery strategy only included areas that contain critical habitat as defined in that document (i.e., those with observations from 1995 to 2018). However, Figure1 and Figure 2 also include hibernacula and swarming sites with observations outside of that time period and sites identified based on new information.
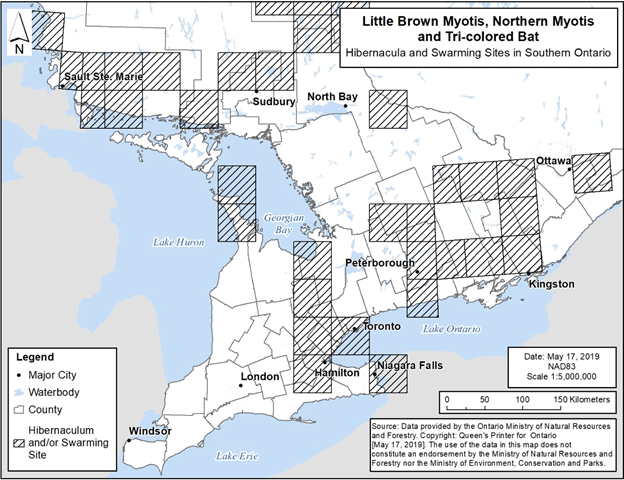
Figure 1. Locations of known hibernacula and swarming sites for Little Brown Myotis, Northern Myotis and Tri-colored Bat in southern Ontario. Each 50 km by 50 km standardized UTM grid square contains at least one hibernaculum and/or swarming site.
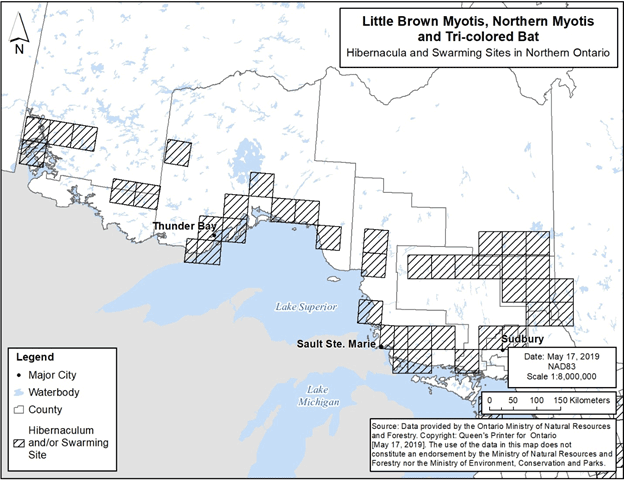
Figure 2. Locations of known hibernacula and swarming sites for Little Brown Myotis, Northern Myotis and Tri-colored Bat in northern Ontario. Each 50 km by 50 km standardized UTM grid square contains at least one hibernaculum and/or swarming site.
Little Brown Myotis
Mobile acoustic ultrasound transect surveys were undertaken by the Ministry of Natural Resources and Forestry (MNRF) province-wide in 2016 and 2017, following the North American Bat Monitoring Protocol (NABat) (Loeb et al. 2015). These surveys identified that Little Brown Myotis was detected with much higher frequency in northwestern Ontario (generally from Thunder Bay westward) than elsewhere in the province in those survey years (Browning and Bowman 2017, M. Browning pers. comm.). In addition, there was a significant decrease in the detection of Little Brown Myotis in 2017 compared to 2016 (Browning and Bowman 2017). This could suggest a widespread decrease in population size in the area corresponding with the expansion front of white-nose syndrome (M. Browning pers. comm.). White-nose syndrome (WNS) was first detected in hibernacula near Thunder Bay in the winter of 2014-15 and has spread westward annually in the winters since (U.S. Fish and Wildlife Service 2018). However, additional research may be needed to firmly establish this potential connection.
Observations of Little Brown Myotis in Ontario to date are well-represented by the map depicting the species’ distribution provided in the federal recovery strategy (ECCC 2018, Figure 1), and this distribution map may be referred to when identifying locations where the species could be encountered. In addition, the specific 50 km by 50 km standardized UTM grid squares containing any observations of Little Brown Myotis in Ontario are shown in Figure 3 and Figure 4 below (NHIC 2019, Jonasson, Guglielmo and Morbey unpub. data, B. Schulte-Hostedde pers. comm., C. Entwistle pers. comm., E. Morris pers. comm., R. Holt pers. comm.). These observations include those that were not able to be visually confirmed; for example, observations made by ultrasonic acoustic recordings.
Areas lacking observations do not represent absence of Little Brown Myotis, rather the absence of data verifying the species’ presence.
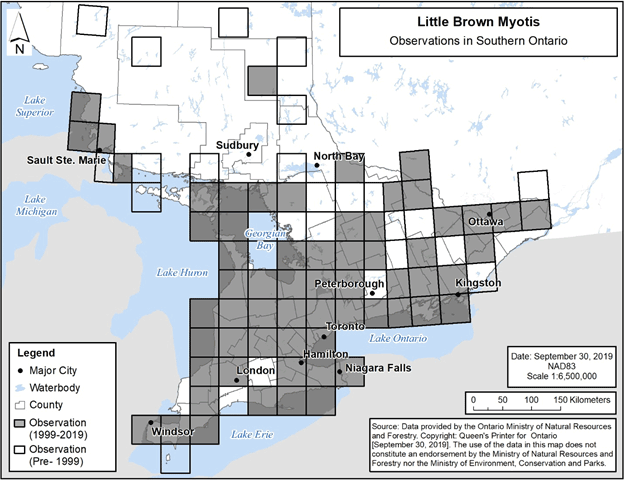
Figure 3. Current (1999-2019) and historic (pre-1999) observations of Little Brown Myotis in southern Ontario. Each 50 km by 50 km standardized UTM grid square contains at least one observation.
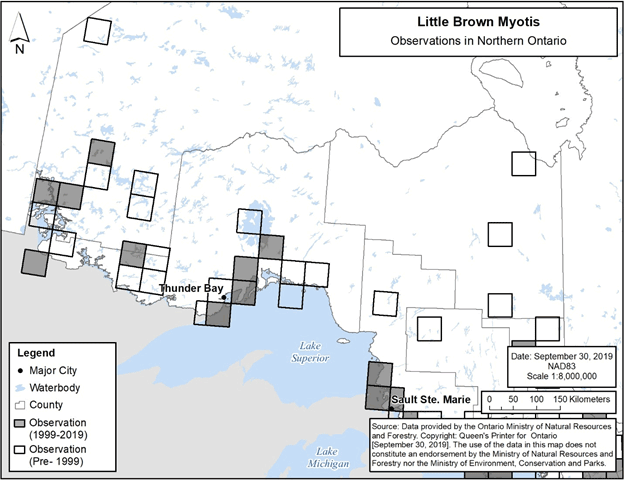
Figure 4. Current (1999-2019) and historic (pre-1999) observations of Little Brown Myotis in northern Ontario. Each 50 km by 50 km standardized UTM grid square contains at least one observation.
Figures 5 and 6 below present occurrence locations for only maternity, foraging and migratory habitat, at a 50 km grid scale. The occurrences displayed in these figures are based on visual confirmation of species identification, i.e., specimens collected, captured, visually examined, or otherwise accepted by the Natural Heritage Information Centre (NHIC) to represent an element occurrence (NHIC 2019, Jonasson, Guglielmo and Morbey unpub. data, B. Schulte-Hostedde pers. comm., C. Entwistle pers. comm., E. Morris pers. comm., R. Holt pers. comm.). Occurrences typically represent locations with multiple observations of a species in a particular year or over multiple years. Element occurrences identified by NHIC were accepted in the applicable category (maternity, foraging or migratory) based on NHIC review of data and/or details included in the record. Element occurrences based on newly available information were identified as maternity occurrences if they were reproductive females, juveniles, or multiple individuals associated with a confirmed roosting feature between May 15 and July 31; migratory occurrences were identified based on records occurring in late summer/early fall or late winter/early spring with either multiple individuals on a given date and no evident association with a particular roosting feature, or individuals in the same location over multiple years.
Figure 5 and Figure 6 present information that adds to what was presented in the federal recovery strategy, as the federal recovery strategy maps depict only the locations of hibernacula and swarming sites.
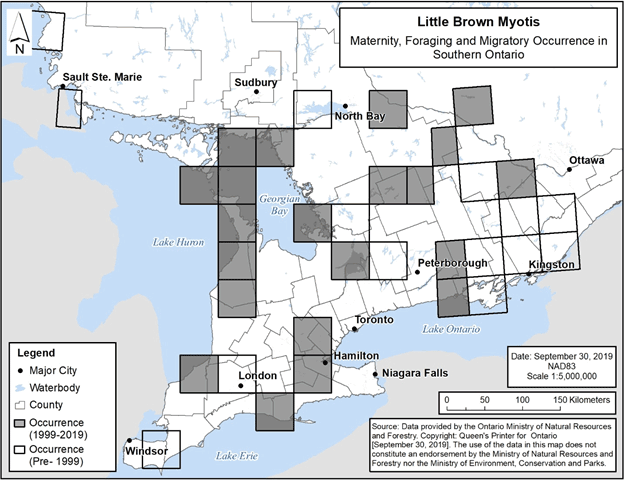
Figure 5. Current (1999-2019) and historic (pre-1999) maternity, foraging and migratory occurrence of Little Brown Myotis in southern Ontario. Each 50 km by 50 km standardized UTM grid square contains at least one element occurrence, i.e., maternity colony, foraging site or location associated with migration.
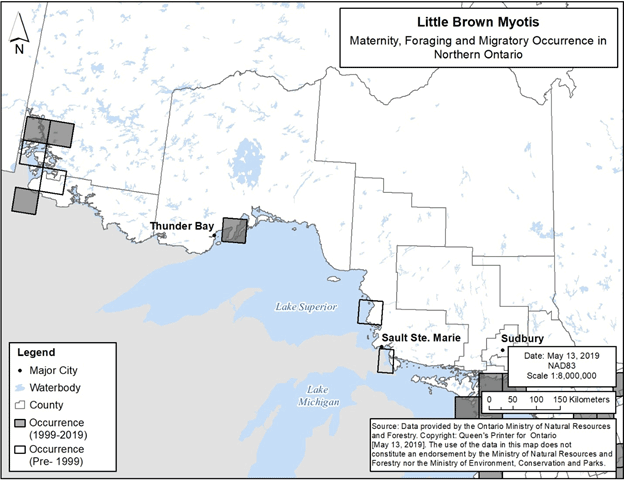
Figure 6. Current (1999-2019) and historic (pre-1999) maternity, foraging and migratory occurrence of Little Brown Myotis in northern Ontario. Each 50 km by 50 km standardized UTM grid square contains at least one element occurrence, i.e., maternity colony, foraging site or location associated with migration.
Areas lacking occurrences do not represent absence of Little Brown Myotis, rather the absence of data verifying the species’ occurrence.
Northern Myotis
Observations of Northern Myotis in Ontario to date are represented by the map depicting the species’ distribution provided in the federal recovery strategy (ECCC 2018, Figure 2), and as a result this distribution map may be referred to when identifying locations where the species could be encountered. In addition, the specific 50 km by 50 km standardized UTM grid squares containing any observations of Northern Myotis in Ontario are shown in Figure 8 below (NHIC 2019, T. Thorne and E. Matczak pers. comm., Jonasson, Guglielmo and Morbey unpub. data). These observations include those that were not able to be visually confirmed; for example, observations made by ultrasonic acoustic recordings.
Areas lacking observations do not represent absence of Little Brown Myotis, rather the absence of data verifying the species’ presence.
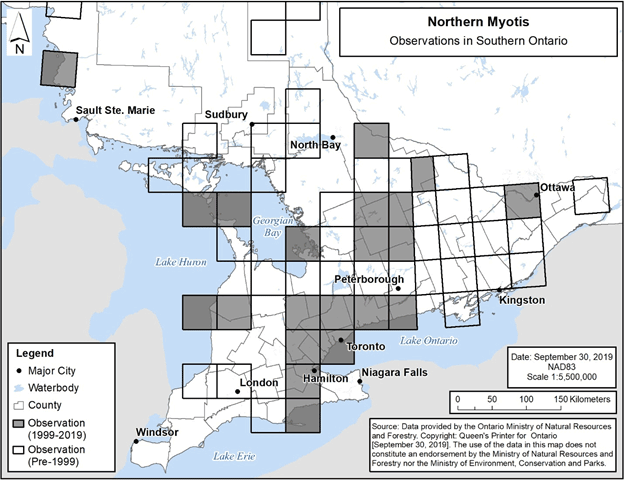
Figure 7. Current (1999-2019) and historic (pre-1999) observations of Northern Myotis in southern Ontario. Each 50 km by 50 km standardized UTM grid square contains at least one observation..
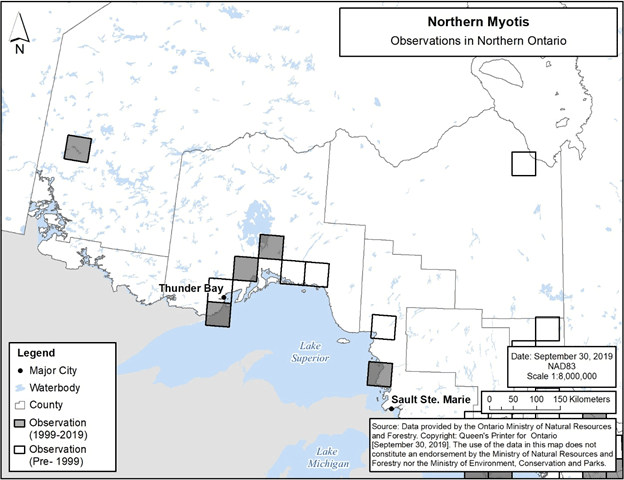
Figure 8. Current (1999-2019) and historic (pre-1999) observations of Northern Myotis in northern Ontario. Each 50 km by 50 km standardized UTM grid square contains at least one observation.
Figure 9 below presents occurrence locations for only maternity, foraging and migratory habitat, at a 50 km grid scale. This figure adds to what was presented in the federal recovery strategy, as the federal recovery strategy maps depict only the locations of hibernacula and swarming sites. The maternity, foraging and migratory occurrences displayed in Figure 7 are based on visual confirmation of species identification, i.e., specimens collected, captured, or visually examined, or otherwise accepted by NHIC to represent an element occurrence (NHIC 2019, T. Thorne and E. Matczak pers. comm., Jonasson, Guglielmo and Morbey unpub. data ). Occurrences typically represent locations with multiple observations of a species in a particular year or over multiple years.
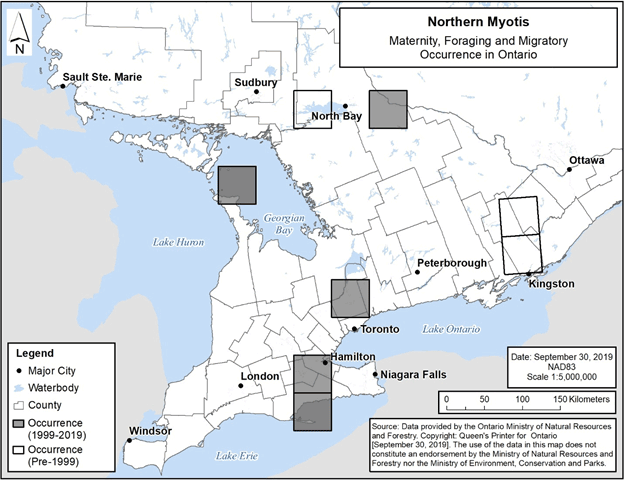
Figure 9. Current (1999-2019) and historic (pre-1999) maternity, foraging and migratory occurrence of Northern Myotis in Ontario. Each 50 km by 50 km standardized UTM grid square contains at least one element occurrence, i.e., maternity colony, foraging site or location associated with migration.
Areas lacking occurrences do not represent absence of Northern Myotis, rather the absence of data verifying the species’ occurrence.
Tri-colored Bat
The specific 50 km by 50 km standardized UTM grid squares containing any observations of Tri-colored Bat in Ontario are shown in Figure 8 below (NHIC 2019, D. Morningstar pers. comm., J. Vandermeulen pers. comm.). These observations include those that were not able to be visually confirmed; for example, observations made by ultrasonic acoustic recordings.
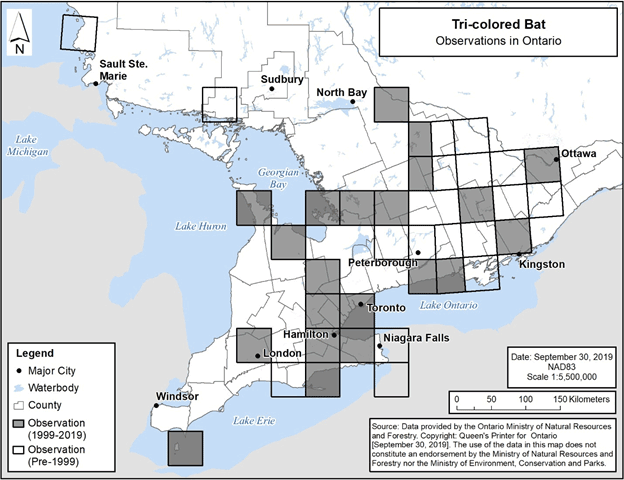
Figure 10. Current (1999-2019) and historic (pre-1999) observations of Tri-colored Bat in Ontario. Each 50 km by 50 km standardized UTM grid square contains at least one observation.
The range limit for Tri-colored Bat in Ontario has traditionally been drawn to encompass an area extending from Essex County in southwestern Ontario to the north end of Algonquin Provincial Park, essentially confined to south and south-central Ontario (Fujita and Kunz 1984, van Zyll de Jong 1985, ECCC 2018). This range is depicted in Figure 11 below.
Most visually confirmed records from Ontario fall within the previously reported range; however, a few records fall outside of it. The furthest north in Ontario that Tri-colored Bat has been visually confirmed was overwintering in a mine on the east shore of Lake Superior; the second-most northern location was overwintering in a mine near the north shore of Lake Huron (NHIC 2019). One recent record also exists from the Bruce Peninsula, which is likewise a hibernation record (D. Morningstar pers. comm.).
Several observations of Tri-colored Bat have also been made north of the range displayed in the federal recovery strategy, all of which were made in Algonquin Provincial Park. These include one occurrence shown on Figure 11.
In addition, new research by Layng et al. (2019) suggests that the range of the Tri-colored Bat may be greater than that indicated in previous range maps, and even greater than accounted for by the visually confirmed records described in the paragraph above. Their study was based on ultrasound acoustic monitoring conducted between 2010 and 2015 as part of the Far North Biodiversity Project. These ultrasound acoustic surveys were undertaken in the Far North of Ontario, that is, “in general, … north of the limits of commercial forestry operations, where abiotic factors limit tree growth rates” (Layng et al. 2019, p. 251). However, further research will be needed to confirm the northern range limit of the species, particularly as their study was not able to produce an accurate distribution map for this species (Layng et al. 2019).
Figure 11 depicts the range map shown in the federal recovery strategy, as well as 50 km grid squares containing hibernacula and swarming sites used by Tri-colored Bat in Ontario, including the three sites described above. It also depicts occurrence locations for maternity, foraging and migratory habitat, at a 50 km grid scale, including the occurrence records north of the range shown in the federal recovery strategy. As a result, this figure adds to what was presented in the federal recovery strategy, as the federal recovery strategy maps only depict the locations of hibernacula and swarming sites. The maternity, foraging and migratory occurrences displayed are based on visual confirmation of species identification, i.e., specimens collected, captured, or visually examined, or otherwise accepted by NHIC to represent an element occurrence (NHIC 2019, D. Morningstar pers. comm., J. Vandermeulen pers. comm.). Occurrences typically represent locations with multiple observations of a species in a particular year or over multiple years. Areas lacking occurrences do not represent the absence of Tri-colored Bat, rather the absence of data verifying the species’ occurrence.
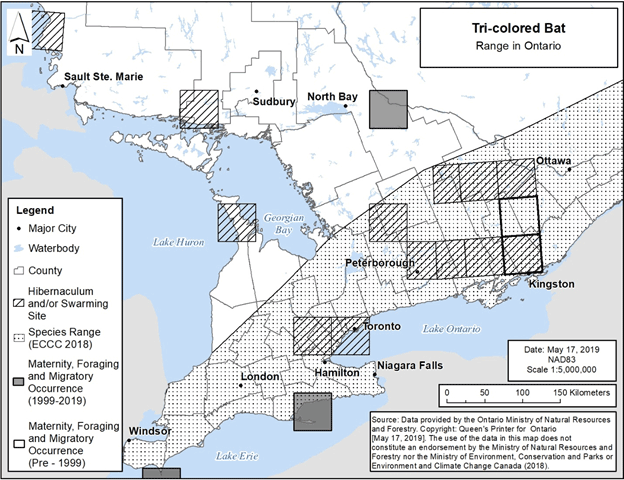
Figure 11. Distribution of the range of Tri-colored Bat in Ontario, with known hibernacula, swarming sites, and maternity, foraging and migratory occurrences. Each 50 km by 50 km standardized UTM grid square contains at least one hibernaculum, swarming site, or element occurrence, i.e., maternity colony, foraging site or location associated with migration.
1.3 Habitat needs
Little Brown Myotis, Northern Myotis and Tri-colored Bat are temperate-zone hibernating bats which employ different behaviours and utilize different habitats to meet their needs seasonally (Lowe 2012, ECCC 2018). The new information on habitat provided below, or information specific to Ontario, is thus described based on seasonal habitat use, including summering habitat (for reproductive females, i.e., maternity habitat, and habitat for males or non-reproductive females) and wintering habitat, i.e., hibernacula and swarming sites. Data or other information on spatial ecology for each of these seasonal habitat types, where available, is also provided to inform the area for consideration in developing a habitat regulation.
Little Brown Myotis
Maternity habitat
Known maternity habitat for Little Brown Myotis in Ontario consists primarily of buildings (NHIC 2019, Heaven 2018) or features associated with buildings, such as artificial roosting structures, and one instance, in between the boards of a raised deck and the bottom of a hot tub (L. Hooton pers. comm., NHIC 2019, Heaven 2018). However, natural roosting sites such as rock crevices, exfoliating tree bark, and cavities and crevices in trees are also known to provide maternity habitat (D. Morningstar pers. comm., Jonasson, Guglielmo and Morbey unpub. data, Natural Resource Solutions Inc. unpub. data).
Little Brown Myotis were captured during a study undertaken between July 12 and July 26, 2017 in a landscape consisting of a mosaic of forests, open woodlands, wetlands and lakes in Ontario (Natural Resource Solutions Inc. unpub. data). Six Little Brown Myotis, including two reproductive adult females and four juveniles were captured in July and tracked to 11 different roost locations using radiotelemetry. Roosts were located in cavities and crevices of live Quaking Aspen (Populus tremuloides), Red Oak (Quercus rubra), Paper Birch (Betula papyrifera) and Pine (Pinus spp.) as well as under exfoliating bark of snags. One of the roosting locations was also located in a bedrock crevice. The minimum area in which roosts were found measured 338 ha in size, calculated by drawing straight lines between the outermost roosts identified. Over 14 days of tracking, 4 of the 11 roosts were used by more than one bat.
A study undertaken in Renfrew County in 2019 identified a variety of tree roosts used by Little Brown Myotis between July 2 and August 21 (R. Holt pers. comm.). Juvenile females, non-reproductive adult females, and adult males were tracked to primarily dead trees (13 of 15) with a minimum diameter at breast height of 21.1 cm. Tree species included, in order of prevalence, Large-tooth Aspen (Populus grandidentata), 53%; Red Maple (Acer rubrum), 13%; and one each (7%) of Black Ash (Fraxinus nigra), Balsam Fir (Abies balsamea), Paper Birch, Quaking Aspen, and a conifer snag (R. Holt unpub. data).
On a roughly 200 ha island in the St. Lawrence River, 53 females in the first two trimesters of pregnancy were captured at their maternity roost and tracked for two to five nights each to identify foraging areas and calculate home range size (Henry et al. 2002). As such, these results are applicable to the home range area, i.e., the roosting and foraging area, used by this colony of Little Brown Myotis during the maternity period. Using fixed-kernel analysis, the 90 percent home range size was 30.1 ± 15.0 ha during pregnancy and 17.6 ± 9.1 ha during lactation (Henry et al. 2002).
Yates et al. (2011) calculated home range sizes for a maternity colony of Little Brown Myotis in a mosaic of forested and agricultural habitats in the Appalachian Mountains of Virginia. A number of Little Brown Myotis were tracked to day roosts and followed by car at night to identify foraging areas for at least three days. As such, these results are applicable to the home range area, i.e., the roosting and foraging area, used by this colony of Little Brown Myotis during the maternity period. Using fixed-kernel analysis and smoothing, the resulting home range size (95th isopleth) for 11 lactating or pregnant female Little Brown Myotis ranged from 90 ha to 8,136 ha in size (mean= 1,780 ha, SE= 687 ha, SD= 2,279 ha) (Yates et al. 2011).
Despite high fidelity to maternity roosting sites, and especially to those in anthropogenic structures, data collected by the MNRF in 2018 identified that reproductive adult females may move between roosts within one maternity season, including those quite far apart (M. Browning pers. comm.). One lactating adult female, which was initially banded at a building maternity roost west of the Greater Toronto Area on June 18, was recaptured three weeks later on July 6 at a different building maternity roost located almost 200 km eastward. While it was initially suspected that the band number may have been read improperly, the same movement had been made by an adult female in the reverse direction just prior, having been banded on June 15 at the same eastern maternity roost and recaptured only three nights later at the same western maternity roost on June 18 (M. Browning pers. comm.).
Male roosting habitat
One male Little Brown Myotis tracked during the summer in Virginia had a calculated home range (95th isopleth) of 661 ha, substantially smaller than the mean size of 1,780 ha for females (Yates et al. 2011).
Thirteen male Little Brown Myotis were tracked by Broders et al. (2006) in the Greater Fundy ecosystem of New Brunswick, which consists of contiguous upland deciduous and lowland/midslope coniferous and mixed forests. They were tracked in summer and into the early swarming season (i.e., early June through late August). Using the 100 percent minimum convex polygon method, the minimum foraging area for males was calculated to be an average of 52.0 ha in size (SD= 57.4 ha). The average distance between successive roosts used was 275 m (SD= 406 m).
As described above, a study undertaken in Renfrew County in 2019 identified a variety of tree roosts used by Little Brown Myotis between July 2 and August 21 (R. Holt pers. comm.). Adult males, non-reproductive adult females and juvenile females were tracked to primarily dead trees (13 of 15) with a minimum diameter at breast height of 21.1 cm. Tree species included, in order of prevalence, Large-tooth Aspen, 53%; Red Maple, 13%; and one each (7%) of Black Ash, Balsam Fir, Paper Birch, Quaking Aspen, and a conifer snag (R. Holt unpub. data).
Hibernacula and swarming sites
In addition to the habitat types described to be used for overwintering in the federal recovery strategy, there is a record of a Little Brown Myotis hibernating in a cold storage room in the basement of a house in Ontario in early March (NHIC 2019).
During the 2014 swarming period on the Bruce Peninsula in Ontario, Jonasson, Guglielmo and Morbey (unpub. data, NHIC 2019) tracked 12 Little Brown Myotis, including adult and juvenile males and females, to roosts located in proximity to known hibernacula. These roosts were located in Quaking Aspen, Paper Birch, Large-tooth Aspen, Balsam Fir, Sugar Maple (Acer saccharum), coniferous and deciduous snags, and in a shed. While these bats were not confirmed to swarm at or enter these nearby hibernacula, tree roosts used in late August were located within 395 to 4,160 m of the nearest known hibernacula (mean= 2,608 m, SE= 415 m, SD= 1,438 m, n= 12 bats, 1 tree roost each). The location of the shed roost is not available.
Lowe (2012) captured adult male Little Brown Myotis at a swarming site in Nova Scotia, and tracked 24 of them to roosts in predominantly coniferous forests used in the area over an average of 3.17 days. These adult males roosted on average within 2,989 m of the swarming site (SD= 2,961 m), but up to a maximum of 13,200 m away. Lowe also identified that both high and low body condition reproductive males roosted in the same proximity to swarming sites; however, low body condition males entered swarming sites/hibernacula more often than high body condition males (Lowe 2012).
Foraging habitat
Jantzen and Fenton (2013) determined that the depth of edge influence for Little Brown Myotis foraging at mixed forests adjacent to hay fields and pastures in eastern Ontario was approximately 40 m; i.e., that activity was highest over the adjacent fields within 40 m of the edge, and over/within the forest within 40 m of the edge. Peak activity for Little Brown Myotis was detected at 8.0 m into the field from the forest edge (Jantzen and Fenton 2013).
Six adult female Little Brown Myotis were identified foraging at sites over 5 km from their maternity roost in southwestern Yukon, while three adult males foraged between 503 to 1,211 m from their summer day roosts (Randall, Jung and Barclay 2014).
Northern Myotis
Maternity habitat
Data on maternity habitat used by Northern Myotis in Ontario is generally lacking. Pregnant or lactating females have been confirmed in roosts in Ontario in one building and in one tree network in the province to date.
Captures of a small number of lactating female Northern Myotis have been made outside of one known building maternity colony for Little Brown Myotis. It may be inferred that these lactating females were roosting in the same building, although it is also possible that they were roosting in adjacent trees (NHIC 2019).
Northern Myotis were captured during a study undertaken by the Toronto Zoo in a large generally forested river valley within the City of Toronto in 2018 (T. Thorne and E. Matczak pers. comm.). In total, five lactating or post-lactating females in addition to three juveniles were captured in July. Through radiotelemetry, three adult female Northern Myotis were tracked over five to thirteen days in July of 2018. Researchers were able to identify a total of five roosts, all within the canopy level of trees, including three roosts in Red Maple and two roosts in Red Oak (Quercus rubra). These roosts were located within approximately 500 m of each other, and all were within 250 m of the river. On two nights, radiotelemetry was undertaken and identified that the tracked Northern Myotis were foraging over the river (Thorne and Matczak unpub. data).
A network of roosts for a maternity colony of Northern Myotis may also exist in one or more buildings and/or trees on the Bruce Peninsula in Ontario (Jonasson, Guglielmo and Morbey unpub. data, NHIC 2019), based on the capture of juvenile Northern Myotis and adults of both sexes in the area. Since captures of juveniles were made no earlier than August 10, which represents a time of year when bats are transitioning to swarming activities (Caceres and Barclay 2000), the status of this roosting network in providing maternity habitat is unclear.
A total of 17 female Northern Myotis were tracked during the maternity and early swarming period in the Greater Fundy ecosystem of New Brunswick. Their minimum foraging area was calculated to be an average of 46.2 ha (SD= 44.4 ha), using the 100 percent minimum convex polygon method (Broders et al. 2006). The distance between successive roosts used by female Northern Myotis averaged 457 m (SD= 329 m).
Henderson and Broders (2008) tracked 21 adult female Northern Myotis belonging to two separate maternity colonies in a mix of agriculture and forested habitat to roosts and foraging areas on Prince Edward Island. The Northern Myotis were tracked for between two to seven days each. Using the 100 percent minimum convex polygon method, the minimum roosting area for each of the colonies was 0.3 ha for a colony which roosted in a forest and then subsequently in a building 435 m away, and 4.13 ha for a colony roosting only in a forest. Overall, the average minimum foraging area (based on 100 percent minimum convex polygon) for 17 Northern Myotis from which foraging data was able to be obtained was 6.09 ha (SD= 5.83). Estimated foraging areas were located over nearby forested creeks; the maximum distances of the foraging areas from the edge of the roosting areas were approximately 730 m and 875 m.
In a similar landscape of agricultural lands and fragmented forests in southern Michigan, 12 Northern Myotis were tracked to maternity roosts in deciduous swamp habitat by Foster and Kurta (1999). The area in which these Northern Myotis were roosting was roughly 20 ha in size.
Owen et al. (2003) tracked 20 pregnant or lactating female Northern Myotis over an average of six days each in intensively-managed hardwood forest in the Appalachian Mountains of West Virginia. Using a 95 percent adaptive kernel method, they calculated home range size for each bat. As such, these results are applicable to the home range area, i.e., the roosting and foraging area, used by this colony of Northern Myotis during the maternity period. Home range sizes ranged from 17.7 to 97.5 ha in size (mean= 63.2 ha, SE= 8.9 ha, SD= 293.6 ha (Owen et al. 2003)).
Thalken, Lacki and Yang (2018) tracked 19 adult female and one juvenile male Northern Myotis between April and July (i.e., between emergence from hibernation and over the maternity period) in Mammoth Cave National Park, Kentucky, a primarily deciduous forested area in karst topography abundant with caves. Distance between successive roosts ranged from 4 to 381 m (mean= 108 m, SE= 12.4 m). They used a spatial point pattern process to describe the locations of observed roosts. Their analysis identified that female Northern Myotis roosted within approximately 500 m of roadways, 800 m of water sources, and within roughly 2,000 to 4,000 m of known bat hibernacula (Thalken, Lacki and Yang 2018). As a result, the authors suggest that a 2,000 m buffer surrounding overwintering caves would be appropriate to protect the availability of roosting sites for female Northern Myotis, particularly in the post-hibernation staging and maternity period.
Male roosting habitat
Minimum foraging areas for 16 male Northern Myotis in New Brunswick were calculated to be 13.5 ha (SD= 8.3 ha) on average, using the 100 percent minimum convex polygon method (Broders et al. 2006). The distance between successive roosts used by male Northern Myotis averaged 158 m (SD= 127 m). Similar to results with home range sizes for Little Brown Myotis estimated by Yates et al. (2011) in Virginia, this calculated minimum foraging area for male Northern Myotis in summer in New Brunswick is substantially smaller than the minimum foraging area calculated for maternity roosting females, which averaged 46.2 ha (Broders et al. 2006). This is also reflected in the observed average distance between successive roosts.
Hibernacula and swarming sites
Although the federal recovery strategy states that Northern Myotis do not typically overwinter in buildings, a hibernaculum consisting of Northern Myotis and Little Brown Myotis was identified in the basement of an unoccupied house on Prince Edward Island (Brown, McAlpine and Curley 2007) and Northern Myotis have been identified hibernating beneath houses built slightly above ground on cinder block props in Nantucket, Massachusetts (Dowling, O'Dell and Beattie 2017).
During the swarming period on the Bruce Peninsula in Ontario, Jonasson, Guglielmo and Morbey (unpub. data, NHIC 2019) tracked four Northern Myotis, including three females and one male, to roosts located in proximity to known hibernacula in late August. These roosts were located in Paper Birch (n= 2), Quaking Aspen (n= 1) and a deciduous snag. While these bats were not confirmed to swarm at or enter these nearby hibernacula, two tree roosts used in late August were located within 210 m and 665 m of one known hibernaculum, and two tree roosts were located within 3,435 m and 3,444 m of another known hibernaculum, respectively (overall mean= 1,939 m).
Lowe (2012) also captured male and female Northern Myotis at a swarming site in Nova Scotia, and tracked six of them (three of each sex) to roosts in predominantly coniferous forests used in the area. The three male Northern Myotis roosted on average within 3,484 m of the swarming site (SD= 2,688 m) and the three female Northern Myotis roosted at a greater distance from the swarming site than males: on average within 4,761 m (SD= 3,720 m).
Foraging habitat
Jantzen and Fenton (2013) determined that the depth of edge influence for Northern Myotis foraging at mixed forests adjacent to hay fields and pastures in eastern Ontario was approximately 40 m; i.e., that activity was highest over the adjacent fields within 40 m of the edge, and over/within the forest within 40 m of the edge. Peak activity for Northern Myotis was detected at 0.8 m into the field from the forest edge (Jantzen and Fenton 2013).
Tri-colored Bat
Maternity habitat
Only two maternity roosts have been identified for Tri-colored Bat in Ontario, both of which were identified in the 1960s and were associated with buildings where maternity colonies of Little Brown Myotis were also found (NHIC 2019).
Maternity habitat for this species elsewhere in its range is also more poorly understood than habitat for Northern Myotis and Little Brown Myotis, but has been identified in the following features:
- Dead leaf clusters in the shape of an umbrella, including dead leaf clusters belonging to broken branches, those formed by natural causes, and from the clusters of dead leaves and other material used in Eastern Gray Squirrel (Sciurus carolinensis) nests (Veilleux, Whitaker Jr. and Veilleux 2003, Davis and Mumford 1962, Findley 1954, Perry and Thill 2007);
- Dense clusters of live foliage (Veilleux, Whitaker Jr. and Veilleux 2003, Perry and Thill 2007);
- Arboreal lichens or epiphytes (Davis and Mumford 1962, Poissant, Broders and Quinn 2010); and
- Buildings, including along outside walls beneath overhangs (e.g., porches, decks) and in garages, sheds and barns (Whitaker 1998).
In a mosaic of mixed coniferous and deciduous forests and various wetland types in Kejimkujik National Park, Nova Scotia, Quinn and Broders (2007) tracked Tri-colored Bats from late May through late August in each of 2003 and 2004. A total of 20 female Tri-colored Bats were tracked to roosts in 60 different trees in seven distinct colonies. During the maternity period, roosts were exclusively located in arboreal lichens (bony bearded lichen, Usnea trichodea). Distance of identified roosts from capture location ranged from 300 m to 5,000 m.
Veilleux et al. (2003) tracked 19 reproductive adult female Tri-colored Bats between late April and July in 1998 and 1999 in a site consisting of a bottomland woods along a creek, riparian woodland, and fragmented upland forests in Indiana. Roosts were identified in foliage clusters in 37 different roost trees representing 14 different tree species. Of the Tri-colored Bats that used at least three roost trees in their maternity roost network (n= 8 individuals), the roosting area was an average of 0.23 ha (SD=0.15 ha, 0.03-0.42 ha). The distance from capture site to the roosting area was available for 15 bats, with a mean of 720 m (SD= 870 m, 50-0.05–2,610 m).
Fifteen adult Tri-colored Bats, including nine reproductive adult females, were also tracked to roosts between May and August of 2015 and 2016 in an area of Kentucky and Tennessee dominated by second-growth oak-hickory forests on a large man-made peninsula (Schaefer 2017). Roosts were typically in dead leaf clusters in a variety of tree species, but predominantly in hickories (Carya spp.) and White Oak (Quercus alba). The distance from capture site to the first roost tree identified averaged 1,120 m (SD=190 m, 165-2,290 m). Roosting areas were defined by a minimum convex polygon for all bats with at least three roost locations identified (n=7 bats) and resulted in minimum roosting areas of 0.79 ha (SD=0.6 ha, 0.002-4.4 ha). Schaefer also reported that Tri-colored Bats in her study were observed foraging in riparian forests (2017).
Male roosting habitat
Yates et al. (2011) calculated home range sizes for male Tri-colored Bat in a mosaic of forested and agricultural habitats in the Appalachian Mountains of Virginia. Tri-colored Bats were tracked to day roosts in June, and followed by car at night to identify foraging areas, for at least three days. Using fixed-kernel analysis and smoothing, the resulting home range size (95th isopleth) for seven male Tri-colored Bats ranged from 76 ha to 957 ha in size (mean= 427 ha, SE= 123 ha, SD= 327 ha, Yates et al. 2011).
Hibernacula and swarming sites
The federal recovery strategy provides evidence that Little Brown Myotis and Northern Myotis often use swarming sites as hibernation sites, and band returns during capture efforts in Ontario have confirmed that this is the case for Tri-colored Bat as well (C. Davy pers. comm.).
1.4 Area for consideration in developing a habitat regulation
Under the ESA, a recovery strategy must include a recommendation to the Minister of the Environment, Conservation and Parks on the area that should be considered in developing a habitat regulation. A habitat regulation is a legal instrument that prescribes an area that will be protected as the habitat of the species. The recommendation provided below will be one of many sources considered by the Minister, including information that may become newly available following completion of the recovery strategy, when developing the habitat regulation for this species.
The Critical Habitat section of the federal recovery strategy provides an identification of some of the critical habitat for these species (as defined under the SARA). Critical habitat was not fully identified in the federal recovery strategy due to a lack of information. Identification of critical habitat is not a component of a recovery strategy prepared under the ESA. However, it is recommended that the approach used to identify the critical habitat in the federal recovery strategy, along with information in this addendum and any new scientific information pertaining to the Little Brown Myotis, Northern Myotis and Tri-colored Bat, and the areas they occupy, be considered when developing a habitat regulation under the ESA.
The following additional recommendations for consideration in the development of a habitat regulation for Little Brown Myotis, Northern Myotis and Tri-colored Bat in Ontario have been derived to inform and support the attainment of the recovery goals for the species as outlined in the federal recovery strategy for the species (ECCC 2018).
Male roosting habitat
Specific early summer roosting habitats for male Little Brown Myotis, Northern Myotis and Tri-colored Bat (i.e., those used after emergence from hibernation in spring until the onset of swarming activities in mid- to late summer) are very challenging to define. While several studies have identified specific roosts used by male Little Brown Myotis, Northern Myotis and Tri-colored Bat, and even identified trends in comparison with maternity roosts at their respective sites, consensus on defining criteria for the characteristics of these roosts at the species level, or characteristic locations in which they occur, has not emerged in the literature (see discussion in Broders et al. 2006, for example). As recommended in the federal recovery strategy, further research into early summer male roosting habitat is included among other research required to fill knowledge gaps to assist in the recovery of these species. As a result, it is recommended that at this time, early summer roosting habitat not be defined in a habitat regulation for male Little Brown Myotis, Northern Myotis or Tri-colored Bat.
Migratory routes and stopover areas
Consistent with the federal recovery strategy, the characteristics and locations of migratory routes and stopover areas for Little Brown Myotis, Northern Myotis and Tri-colored Bat, and the extent to which they contribute to the ability of each species to carry on its’ life processes, are generally unknown. As a result, it is recommended that at this time, migratory routes and/or stopover areas not be included within a habitat regulation for Little Brown Myotis, Northern Myotis or Tri-colored Bat.
Foraging habitat
Several specific areas are known to provide foraging habitat for Little Brown Myotis, Northern Myotis and Tri-colored Bat in Ontario, and the habitat types in which these species can be found foraging are generally understood; however, they are very broadly described, widespread, and extensive in the province (e.g., wetlands, forests and forest edges, etc.). Since it is understood that these species require foraging areas within a reasonable commuting distance of concentration areas such as maternity roosts and swarming sites (Lowe 2012, ECCC 2018), sufficient area to allow appropriate foraging resources during critical life cycle periods is included within the recommended areas for habitat regulation as outlined below. This is incorporated by relying on data pertaining to home ranges, minimum foraging areas, or specific distances known to be travelled by bats in relation to these habitat types, to ensure that adequate foraging habitat is protected.
Hibernacula and swarming sites
The federal recovery strategy identified hibernacula to provide critical habitat for Little Brown Myotis, Northern Myotis and Tri-colored Bat (ECCC 2018). It is recommended that the definition and supplementary criteria provided in that document orm part of the consideration for the identification of hibernacula as habitat in a habitat regulation. These are:
- “Any site where hibernation by Little Brown Myotis, Northern Myotis and/or Tri-colored Bat has been observed at least once between 1995 and 2018” (pg. 74);
- “other forms of evidence of occupancy can be used (e.g., acoustic data, genetic material, swarming behaviour)” to identify hibernacula (pg. 75);
- “bat activity in the spring during the period when bats emerge from hibernacula may also be used as an indicator of the sites’ occupancy” (pg. 75); and
- “A threshold number of individuals is not defined in the occupancy criterion due to the difficulty in locating individuals within hibernacula and obtaining accurate counts, while also recognizing that the discovery of one hibernating individual often indicates the occurrence of more undetected individuals using the hibernaculum” (pg. 75).
Additional details of these criteria can be found in the federal recovery strategy.
Swarming activity consists of congregations of bats flying in circles at the entrance to hibernacula, or in and out of a hibernaculum or potential hibernation feature, when mating occurs before bats prepare to hibernate (Fenton 1969). As the federal recovery strategy noted, some bat swarming sites are not sites of hibernation; however, swarming sites can often be indicative of a hibernaculum or at a minimum, are important sites for gene flow during mating. Because of the importance of both site types and their associated activities, as well as the difficulty in identifying smaller hibernacula which may still support this important life process for these species, it is recommended that both swarming and hibernation sites should be prescribed as habitat in a habitat regulation, in their own right.
It is recommended that, until knowledge gaps are addressed, the following criteria be used in addition to those outlined in the federal recovery strategy to identify regulated habitat for hibernation and swarming:
- It is recommended that the basis for identifying a hibernaculum or swarming site should be the presence of one or more Little Brown Myotis, Northern Myotis and/or Tri-colored Bat or their genetic material within, at, or within 30 m of the entrance to a cave, abandoned mine, well or tunnel, or any other site which may provide suitable environmental conditions (i.e., stable, cool, humid climate as described in the federal recovery strategy), as documented between August 1 and May 15 (Fenton and Barclay 1980, Fujita and Kunz 1984, Caceres and Barclay 2000, ECCC 2018). The use of a 30 m distance from the entrance to a site is based on the approximate range of an ultrasonic acoustic detector which may be placed within 10 m of the entrance to a potential hibernation or swarming site. Rationale: This recognizes that population sizes at hibernacula may fluctuate within and between years, and may be as low as zero individuals detected in a given year.
- All hibernacula and swarming sites for Little Brown Myotis, Northern Myotis and Tri-colored Bat that have been used at any point in the past are recommended to be prescribed as habitat in a habitat regulation. It is recommended to include hibernacula or swarming sites in natural or anthropogenic structures, unless a site is no longer suitable (e.g., structure collapsed or destroyed, access blocked to prevent bats from entering, permanently flooded), or is considered to be occupied (i.e., resided in) by humans. Rationale: This recognizes that these species “exhibit strong site fidelity to hibernacula, individual sites may be used by hibernating bats for decades, and suitable hibernacula may be limiting” (ECCC 2018). It also acknowledges that all hibernacula may not have been surveyed at least once in the last 23 years, and available information suggests that hibernacula may continue to be used while conditions remain suitable at the site.
- It is recommended that roosting and foraging resources within 2,600 m of a hibernaculum and/or swarming site be identified as habitat in the habitat regulation because bats typically roost outside of, but near, hibernacula during the swarming and post-hibernation period. Rationale: This recommended area for roosting and foraging resources near hibernacula/swarming sites was derived from the following data:
- The recommended distance of 2,600 m represents the rounded average distance that 12 Little Brown Myotis, including juveniles and adults of both sexes, were roosting in proximity to the nearest hibernacula during the swarming season (late August) in Ontario (Jonasson, Guglielmo and Morbey unpub. data, NHIC 2019).
- The average distance that 24 adult male Little Brown Myotis roosted from their known swarming site in Nova Scotia was similar to (although slightly larger than) the recommended distance, at 2,989 m (Lowe 2012).
- The average distance that four Northern Myotis were roosting in proximity to the nearest hibernacula during the swarming season in Ontario was 1,939 m (Jonasson, Guglielmo and Morbey unpub. data, NHIC 2019).
- The average distances that three male Northern Myotis and three female Northern Myotis were roosting in proximity to their known swarming sites in Nova Scotia were larger, at 3,484 m and 4,761 m, respectively (Lowe 2012).
- It is presumed that foraging may occur within a minimum distance between the hibernacula/swarming site and nearby roosts.
- Foraging resources within a reasonable travelling distance from a hibernaculum are important as bats must be in prime body condition when entering hibernation in fall, with the fall swarming season representing a period of fat accumulation, and are in poor body condition when exiting hibernation in spring (Lowe 2012).
The use of 2,600 m represents an intermediate value in the range of information available to date. Average distances available for Northern Myotis roosts near hibernacula are both less than, and greater than, the average distances for Little Brown Myotis roosts (1,939 m in Ontario, and 3,484 m and 4,761 m for males and females, respectively, in Nova Scotia). Maximum distance values have not been recommended as it is unknown whether maximum distances represent outliers due to the lack of comprehensive studies available. The distances that Tri-colored Bats roost from their hibernacula or swarming sites are not available. Despite this, the species’ general ecology and life cycle are similar to those of Little Brown Myotis and Northern Myotis (i.e., they are likewise a temperate hibernating bat species that makes short-distance migrations, moving between early summer maternity and roosting sites, and late summer/fall swarming and hibernation sites), so these resources are assumed to be relied upon for this species as well. The types of roosting and foraging habitats recommended to be protected are described in points 4 and 5 below. The area within 2,600 m of a hibernaculum or swarming site is equivalent to a 2,123 ha area based on a point centroid with a circular buffer.
- It is recommended that the 2,600 m distance extend from all known or suspected entrances of a hibernaculum or the total underground extent of a hibernaculum, if known, and/or the concentrated area of swarming activity. If one entrance is known, the entrance should represent a point in the center of a circle with a radius of 2,600 m. If multiple entrances are known, but the full underground extent of the site is not known, a polygon should be drawn between the entrance locations, using straight lines, to identify the hibernaculum site. For sites with multiple entrances, or where the underground extent of a hibernaculum is known, foraging and roosting resources within an area extending 2,600 m from the boundary of the known hibernaculum polygon should be included in the habitat. Rationale: This approach protects the hibernaculum as the core feature of the habitat, supported by adequate fall and spring roosting sites and foraging resources in proximity to the hibernaculum. This recognizes that information on the extent of caves or abandoned mines and how conditions within them (e.g., temperature, humidity and air flow) may be affected by nearby surface or ground activities is limited.
- Specific roosting features that are recommended to be protected within 2,600 m of the hibernaculum or swarming site include trees (with exfoliating or raised bark, cavities, crevices, or umbrella-shaped clumps of foliage), snags, stumps, rocky habitats (both natural, such as cliff, talus and barrens, and anthropogenic, such as rip-rap) and structures unoccupied by humans (i.e. in which humans do not reside) such as bridges or concrete box culverts, barns, sheds and empty houses.
- It is recommended that natural communities around hibernacula or swarming sites be described based on the Ecological Land Classification (ELC) methods for southern Ontario (Lee et al. 1998) or ELC methods for the Far North, the Boreal, the Great Lakes-St. Lawrence, or the South (Operational Drafts or their successors, as applicable and as they may become available) (Banton et al. 2009). The following foraging resources within 2,600 m of the hibernaculum or swarming site should be protected: forests, wetlands and waterbodies (e.g., FO, SW, FE, Bog, MA, OA, SA Community Classes or CUW, ALT, RBT, SBT, TAT, TPW Community Series using ELC for southern Ontario, as well as creeks, streams, ponds and other water bodies that may not otherwise be classified per ELC). The following should also be protected as foraging resources: the extent of meadows, thickets, savannahs and old fields (e.g., CUM, CUT, CUS Community Series, per ELC for southern Ontario) that are within 40 m of forests (i.e., FO, SWD, SWM, SWC, RBT, SBT, TAT, TPW) up to 2,600 m from hibernaculum/swarming site. Rationale: Ecological Land Classification is recommended in order to standardize the application of habitat protection.
- Foraging and roosting features are recommended to be protected regardless of their contiguity to the hibernaculum/swarming site. Rationale: Little Brown Myotis, Northern Myotis, and Tri-colored Bat are each highly mobile, and utilize airspace above unsuitable habitats for travelling to foraging and roosting resources. Specific data on foraging resources used by these species in proximity to their hibernacula or swarming sites is not available. However, based on movements of bats observed during tracking studies, they may be found in close proximity to hibernacula or at much greater distances than roosts have been documented (Lowe 2012). Foraging and roosting resources falling within 2,600 m are therefore recommended to be protected due to the importance of energetics and body condition to bats entering hibernation.
Maternity sites
Maternity sites are relied upon by female Little Brown Myotis, Northern Myotis and Tri-colored Bat in the spring and early summer, not long after emerging from hibernation, when they are recuperating from poor body condition. Females, and typically some males, are likely to congregate in suitable sites with sufficient protection from predators, abundance of roosting locations and adequate solar exposure to achieve appropriate body temperatures (ECCC 2018).
It is recommended that, until knowledge gaps are addressed, the following criteria be used to identify regulated habitat relating to the maternity period for Little Brown Myotis, Northern Myotis and Tri-colored Bat:
- Maternity sites for Little Brown Myotis, Northern Myotis and Tri-colored Bat are recommended to be identified based on any location where an observation of a roosting pregnant or lactating female, juvenile female, or juvenile male has been made between May 15 and July 31 (Fenton and Barclay 1980, Fujita and Kunz 1984, Caceres and Barclay 2000, ECCC 2018) and the individual is observed in or observed to exit from an identifiable roosting feature, e.g., a tree, rock feature or an unoccupied structure (i.e., a structure not resided in by humans). In the event that the sex or age of roosting individuals cannot be determined, maternity sites may also be identified based on any feature where two or more Little Brown Myotis, Northern Myotis or Tri-colored Bat are observed in or observed to exit from the feature between May 15 and July 31. Sufficient data are currently lacking to differentiate arrival and departure dates from maternity sites in different regions of the province at this time.
- It is recommended that maternity sites in natural communities (e.g., roosts of any type in forests) and their surrounding natural areas be described based on the ELC methods for southern Ontario (Lee et al. 1998) or ELC methods for the Far North, the Boreal, the Great Lakes-St. Lawrence, or the South (Operational Drafts or their successors, as applicable and as they may become available) (Banton et al. 2009). Maternity sites in natural communities should be identified to at least the ecosite level. Rationale: Ecological Land Classification is recommended in order to standardize the application of habitat protection.
- The contiguous ecosite containing roosting Little Brown Myotis, Northern Myotis or Tri-colored Bat, to a maximum area as outlined for each species below, should be included as the regulated maternity habitat site. The boundaries of the roosting area may be further refined if sufficient information is available to clearly refine the core roosting area used (i.e., through a radio-telemetry study). Rationale: The ecosite is recommended to be identified as the maternity habitat site as it is unlikely that all or most roosts used by individuals in a maternity colony will be identified without the use of radiotelemetry or other tracking methods. This approach recognizes that these species tend to be highly mobile, change roost sites frequently within an area, and that the likelihood of detecting all specific roosts being used is low.
- For anthropogenic sites such as buildings, bridges, rip-rap and quarries, it is recommended that the contiguous area providing the same type of roosting habitat used by the species should be identified as the regulated maternity habitat site. Examples are: an entire bridge, a building, a contiguous extent of rip-rap, a cut rock face, or a rock pile. In addition, alternate roost sites used (e.g., nearby trees or other roosts that are used less frequently than a primary anthropogenic site) are also recommended to be identified as regulated maternity habitat. Each alternate roost site should be treated as an individual maternity habitat site for the purposes of applying the habitat regulation. This includes alternate roosts within a forested natural community per ELC, in anthropogenic sites, and roosts in individual features such as trees or rocks (i.e., those not associated with a natural community per ELC). If alternate roosts used by an anthropogenic maternity colony are found within a natural community per ELC, the portion of contiguous ecosite in which the roosting occurs should be identified as a maternity habitat site as well, per point 3 above.
- The observation of Little Brown Myotis, Northern Myotis, or Tri-colored Bat within caves or abandoned mines between May 15 and July 31 should not constitute identification of a maternity site, since caves and abandoned mines do not provide maternity habitat for these species in Ontario. Also, anthropogenic objects such as vehicles or mobile homes that could be easily moved should not be considered maternity habitat.
- It is recommended that all maternity sites identified to date in Ontario, regardless of date of last observation or study, should be identified as habitat in a habitat regulation, unless the habitat is no longer suitable (e.g., natural community has since been removed; structure has collapsed or been destroyed; access now blocked to preclude bats from entering), or the species is no longer present at the site during the maternity period. Rationale: This addresses the high degree of fidelity of these species to maternity roost sites (ECCC 2018) and assumes a precautionary approach in the event that natural roosting sites have not recently been studied.
It is also recommended that the following additional criteria be used to identify regulated habitat relating to the maternity period for Little Brown Myotis:
- Based upon ELC completed at the maternity site:
- It is recommended that in the case of maternity roosting in a natural community, the contiguous ecosite for a maternity site for Little Brown Myotis should be no greater than 1,800 ha. A large ecosite of this size is more likely to occur in northern Ontario habitats where large contiguous forests may be found.
- In most cases, particularly in southern Ontario, in highly diverse landscapes, or highly fragmented landscapes, the contiguous ecosite will be much smaller than 1,800 ha. In cases where the contiguous ecosite is smaller than 1,800 ha, and in the case of anthropogenic roosting features, it is recommended that an area including the ecosite or roosting feature plus surrounding foraging resources (described in point 8 below) are included as habitat in a habitat regulation. Rationale: This recognizes the importance of ensuring adequate foraging and drinking water resources are available for lactating adult females and juvenile bats in areas with more limited resources available on the landscape.
- In total, this area of foraging resources is recommended to extend no more than 2,400 m beyond the boundary of a maternity site, or to a maximum of 1,800 ha. This 2,400 m distance from the maternity site represents roughly a 1,800 ha area based on a point centroid with a circular buffer. Rationale: This area is based on and approximates the mean home range size (i.e., roosting and foraging area) identified for pregnant and lactating female Little Brown Myotis during the maternity period in Virginia (Yates, et al. 2011). The mean home range size from Virginia (Yates, et al. 2011) forms the basis of the recommended area to be protected for foraging Little Brown Myotis during the maternity period, rather than the data available from Quebec (Henry, et al. 2002), as the colony’s activity in Quebec could have been constrained by the small size and isolation of its island location in the St. Lawrence River, and thus may not be representative of most maternity colonies in Ontario. Data on the distance of a known and frequented foraging site for reproductive females in southwestern Yukon (i.e., >5,000 m from the maternity roost site, Randall, Jung and Barclay 2014) also supports a larger area more consistent with that identified in the study from Virginia.
- Maximum home ranges have not been recommended as it is unknown whether maximum home ranges represent outliers, as there are few comprehensive studies available to date.
- The minimum area containing roosts identified in the study undertaken by Natural Resource Solutions Inc. (338 ha, unpub. data) was not used to identify the recommended area to be protected for foraging Little Brown Myotis during the maternity period as it represents only the roosting area, and data on the foraging area for this colony is not available.
- The area of the foraging resources recommended to be protected may be further refined if sufficient information is available to rule out the use of habitat within this area for foraging (e.g., through a detailed ultrasonic acoustic monitoring or radio-telemetry study).
- Potential foraging resources within 2,400 m of the maternity site (in the case of contiguous maternity sites that are smaller than 1,800 ha) are recommended to be identified as regulated habitat. Foraging resources recommended to be protected include:
- Forests, wetlands and waterbodies (e.g., FO, SW, FE, Bog, MA, OA, SA Community Classes or CUW, ALT, RBT, SBT, TAT, TPW Community Series using ELC for southern Ontario),
- Creeks and streams that may not otherwise be classified per ELC, and
- The extent of meadows, thickets and savannahs or other old fields (e.g., CUM, CUT, CUS Community Series, per ELC for southern Ontario) that are within 40 m of forests or woodlands (i.e., FO, SWD, SWM, SWC, CUW, ALT, RBT, SBT, TAT, TPW per ELC for southern Ontario). Rationale: This criterion acknowledges that foraging activity for Little Brown Myotis has been found to be highest within 40 m of woodland edges (Jantzen and Fenton 2013).
- These foraging resources are recommended to be protected regardless of their contiguity to the roosting site. Rationale: Little Brown Myotis is highly mobile and utilizes airspace above unsuitable habitats for travelling to foraging and roosting resources. The movements of bats observed during tracking studies identify home range sizes that include primary foraging locations. Foraging resources falling within 2,400 m are therefore recommended to be protected due to the importance of body condition to pregnant and lactating females and juveniles.
It is recommended that the following additional criteria be used to identify regulated habitat relating to the maternity period for Northern Myotis:
- Based upon ELC completed at the maternity site:
- It is recommended that in the case of maternity roosting in a natural community, the contiguous ecosite for a maternity site for Northern Myotis should be no greater than 63 ha. A large ecosite of this size is more likely to occur in northern Ontario habitats where large contiguous forests may be found.
- In most cases, particularly in southern Ontario, in highly diverse landscapes, or highly fragmented landscapes, the contiguous ecosite will be much smaller than 63 ha. In cases where the contiguous ecosite is smaller than 63 ha, and in the case of anthropogenic roosting features, it is recommended that an area including the ecosite or roosting feature plus foraging resources (described in point 10 below) be included as habitat in a habitat regulation. Rationale: This recognizes the importance of ensuring adequate foraging and drinking habitat resources are available for lactating adult females and juvenile bats in areas with more limited resources available on the landscape.
- In total, this area of foraging resources is recommended to extend no more than 450 m beyond the boundary of a maternity site. The 450 m distance from the maternity site approximates a 63 ha area based on a point centroid with a circular buffer. Rationale: This area is based on the mean home range size from a maternity colony in West Virginia (Owen, et al. 2003) as this represents the most comprehensive information available on the area of habitat relied upon for maternity roosting Northern Myotis. The average minimum foraging area that was identified for females during the maternity period in New Brunswick (46.2 ha, Broders, et al. 2006) represents a different metric of habitat use than an average home range size, and is by nature smaller than a home range. However, this minimum foraging area also suggests that the home range size for this species in New Brunswick may be similar to that documented in West Virginia.
- The maximum observed home range has not been recommended as it is unknown whether the maximum home range represents an outlier as a result of few comprehensive studies available to date.
- The area of the foraging resources recommended to be protected may be further refined if sufficient information is available to rule out the use of habitat within this area for foraging (e.g., through a detailed ultrasonic acoustic monitoring or radio-telemetry study).
- Potential foraging resources within 450 m of the maternity site (in the case of contiguous maternity sites that are smaller than 63 ha) are recommended to be identified as regulated habitat. Foraging resources recommended to be protected for Northern Myotis within this area include:
- Forests, wetlands, and waterbodies (e.g., FO, SW, FE, Bog, MA, OA, SA Community Classes or CUW, ALT, RBT, SBT, TAT, TPW Community Series using ELC for southern Ontario),
- Creeks and streams that may not otherwise be classified per ELC, and
- The extent of meadows, thickets and savannahs or other old fields (e.g., CUM, CUT, CUS Community Series, per ELC for southern Ontario) that are within 40 m of forests (i.e., FO, SWD, SWM, SWC, CUW, ALT, RBT, SBT, TAT, TPW).
- These are recommended to be protected regardless of their contiguity to the roosting site. Rationale: Northern Myotis is highly mobile and utilizes airspace above unsuitable habitats for travelling to foraging and roosting resources. The movements of bats observed during tracking studies identify home range sizes that include primary foraging locations. Foraging resources falling within 450 m are therefore recommended to be protected due to the importance of body condition to pregnant and lactating females and juveniles.
It is also recommended that the following additional criteria be used to identify regulated habitat relating to the maternity period for Tri-colored Bats:
- Based upon ELC completed at the maternity site:
- It is recommended that in the case of maternity roosting in a natural community, the contiguous ecosite for a maternity site for Tri-colored Bat should be no greater than 265 ha. A large ecosite of this size is more likely to occur in northern Ontario habitats where large contiguous forests may be found.
- In most cases, particularly in southern Ontario, in highly diverse landscapes, or highly fragmented landscapes, the contiguous ecosite will be much smaller than 265 ha. In cases where the contiguous ecosite is smaller than 265 ha, and in the case of anthropogenic roosting features, it is recommended that an area including the ecosite or roosting feature plus foraging resources (described in point 12 below) be included as habitat in a habitat regulation. Rationale: This recognizes the importance of ensuring adequate foraging and drinking habitat resources are available for lactating adult females and juvenile bats in areas with more limited resources available on the landscape.
- In total, this area of foraging resources is recommended to extend no more than 920 m beyond the boundary of a maternity site. The 920 m distance from the maternity site approximates a 265 ha area based on a point centroid with a circular buffer. Rationale: This area is based on the mean distance traveled from capture site to roosting networks from maternity colonies in Indiana and Kentucky (Veilleux, Whitaker and Veilleux 2003, Schaefer 2017) as these represent the most comprehensive information available on the average distance that Tri-colored Bats might travel between roosting areas and foraging areas (assuming that Tri-colored Bats were captured while foraging, as a minimum). The distance of 920 m represents the mid-point between the mean distances travelled between capture sites and roosting locations reported in each study (720 m and 1,120 m, respectively).
- The maximum travelling distance observed between capture sites and identified roosts or roost areas has not been used as the basis for the recommendation as it is unknown whether or how many of the captured bats were foraging in their typical home range or if the maximum values reported in the available studies represent outliers.
- The area of the foraging resources recommended to be protected may be further refined if sufficient information is available to rule out the use of habitat within this area for foraging (e.g., through a detailed ultrasonic acoustic monitoring or radio-telemetry study).
- Potential foraging resources within 920 m of the maternity site (in the case of contiguous maternity sites that are smaller than 265 ha) are recommended to be identified as regulated habitat. Foraging resources recommended to be protected for Tri-colored Bat within this area include:
- Riparian forests, i.e. those adjacent to rivers, streams, ponds or lakes (e.g. FO, SW, Community Classes or CUW, ALT, RBT, SBT, TAT, TPW Community Series using ELC for southern Ontario, where they occur adjacent to, or contain, watercourses or water bodies). Where floodplains and/or bottomlands occur in association with watercourses or water bodies, the foraging habitat should be restricted to those fresh-moist woodlands or forests (e.g. fresh-moist CUW Community Series or FOC3, FOC4, FOM6, FOM7, FOM8, FOD6, FOD7, FOD8 Ecosites using ELC for southern Ontario), or treed swamps (SWC, SWM, SWD) occurring in the floodplain/bottomlands. Where floodplains and/or bottomlands do not occur in association with watercourses or water bodies, the foraging resource should be restricted to the forested Ecosite immediately adjacent to or containing the watercourse or water body,
- Wetlands and water bodies (e.g., SW, FE, Bog, MA, OA, SA Community Classes using ELC for southern Ontario),
- Creeks and streams that may not otherwise be classified per ELC or which lack adjacent forest cover, and
- Meadows, thickets and savannahs or other old fields (e.g., CUM, CUT, CUS Community Series, per ELC for southern Ontario), based on general identification of open areas as suitable foraging habitat (ECCC 2018, Ethier and Fahrig 2011).
- These are recommended to be protected regardless of their contiguity to the roosting site. Rationale: Tri-colored Bats are highly mobile and can utilize airspace above unsuitable habitats for travelling to foraging and roosting resources. Foraging resources falling within 920 m are therefore recommended to be protected due to the importance of body condition to pregnant and lactating females and juveniles.
Figures 12 and 13 below provide illustrative examples of the habitat protection that is recommended to be applied to a maternity roost for Tri-colored Bat in an anthropogenic structure, and a maternity roost tree network for Northern Myotis which occurs in a natural forested habitat, respectively. While these illustrative examples focus on maternity habitat protection for Tri-colored Bat and Northern Myotis, the approaches are also applicable to maternity habitat for Little Brown Myotis and to hibernacula/swarming habitats for all three species.
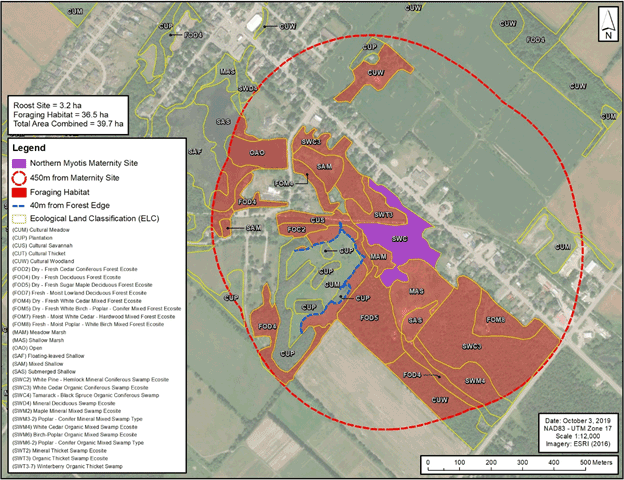
Figure 12. Illustrative example of the recommended area to be identified as maternity habitat for Northern Myotis tracked to roost trees in a forested habitat. This example identifies the maternity site as the contiguous ecosite where the trees hypothetically used as maternity roosts by a colony of Northern Myotis are located. It also identifies the associated foraging resources in the surrounding area not exceeding 450 m from the boundary of the ecosite containing the roost trees, nor exceeding 63 ha of combined area in total (roosting ecosite plus foraging resources). Both the maternity site (ecosite) and the surrounding foraging resources are recommended to be protected as regulated habitat under the ESA.
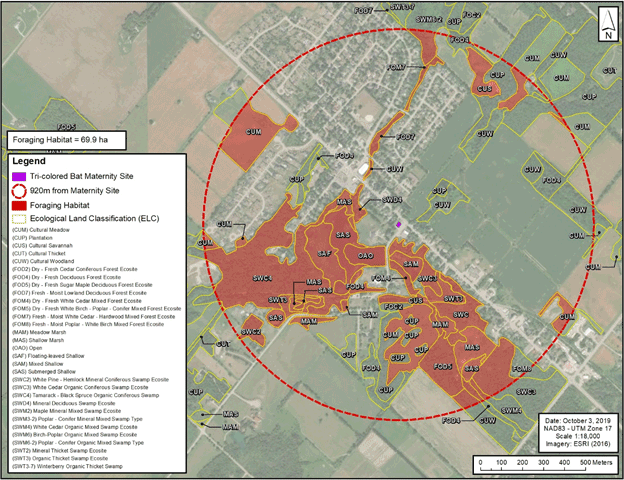
Figure 13. Illustrative example of the recommended area to be identified as maternity habitat for Tri-colored Bat utilizing an anthropogenic structure. This example identifies a hypothetical anthropogenic structure used as a maternity roost by a colony of Northern Myotis as the maternity site, and the associated foraging resources up to 920 m from the structure. Both the maternity site and the surrounding foraging resources are recommended to be protected as regulated habitat under the ESA.
Summary
The following represents a summary of the above recommended area for consideration in developing a habitat regulation for Little Brown Myotis, Northern Myotis, and Tri-colored Bat:
Foraging habitat: Foraging habitat is important for multiple life stages and processes and is therefore included within habitat recommendations for maternity roosts, hibernacula and swarming sites.
Hibernacula and swarming sites: It is recommended that all known hibernacula and swarming sites for Little Brown Myotis, Northern Myotis and/or Tri-colored Bat be prescribed as habitat in a habitat regulation, with the exception of any sites which are no longer suitable (e.g., structure collapsed or destroyed, access blocked to prevent bats from entering, permanently flooded) and any anthropogenic structures resided in by humans. It is further recommended that foraging and roosting resources within 2,600 m of a hibernaculum and/or swarming site be identified as habitat in the habitat regulation. The area should extend 2,600 m from all known or suspected entrances of a hibernaculum, or total underground extent of a hibernaculum, if known, and/or the concentrated area of swarming activity.
Maternity sites: For Little Brown Myotis, Northern Myotis and Tri-colored Bat, it is recommended that maternity habitat be identified based on the contiguous ecosite or contiguous anthropogenic site where all known observations of roosting adult females and juveniles between May 15 and July 31 have been made, unless the habitat is no longer suitable, or bats are no longer roosting at the site. Maternity sites may also be identified based on any feature where two or more Little Brown Myotis, Northern Myotis or Tri-colored Bat have been observed in, or observed to exit from, the feature, between May 15 and July 31. It is further recommended that foraging areas within 2,400 m of the boundary of a maternity site for Little Brown Myotis, 450 m of the boundary of a maternity site for Northern Myotis, and 920 m of the boundary of a maternity site for Tri-colored Bat be identified as supporting foraging habitat in the habitat regulation. The combined roosting and foraging habitat should not exceed 1,800 ha, 63 ha, and 265 ha in total, respectively.
Future research on the roosting, migratory, foraging and maternity habitats used by Little Brown Myotis, Northern Myotis and Tri-colored Bat, as recommended in the federal recovery strategy, should be used to re-assess the suitability of the area recommended for inclusion in habitat regulations for these species, and to update the habitat regulations for these species as needed.
Glossary
- Committee on the Status of Endangered Wildlife in Canada (COSEWIC)
- The committee established under section 14 of the Species at Risk Act that is responsible for assessing and classifying species at risk in Canada.
- Committee on the Status of Species at Risk in Ontario (COSSARO)
- The committee established under section 3 of the Endangered Species Act, 2007 that is responsible for assessing and classifying species at risk in Ontario.
- Conservation status rank
- A rank assigned to a species or ecological community that primarily conveys the degree of rarity of the species or community at the global (G), national (N) or subnational (S) level. These ranks, termed G-rank, N-rank and S-rank, are not legal designations. Ranks are determined by NatureServe and, in the case of Ontario’s S-rank, by Ontario’s Natural Heritage Information Centre. The conservation status of a species or ecosystem is designated by a number from 1 to 5, preceded by the letter G, N or S reflecting the appropriate geographic scale of the assessment. The numbers mean the following: 1 = critically imperilled
2 = imperilled
3 = vulnerable
4 = apparently secure
5 = secure
NR = not yet ranked - Endangered Species Act, 2007 (ESA)
- The provincial legislation that provides protection to species at risk in Ontario.
- Epiphyte
- An organism (typically a plant) which grows on the surface of another plant for support, and derives its nutrients and moisture from the environment.
- Extant (element occurrence)
- An area of land or water where a species is still in existence.
- Hibernaculum (pl. hibernacula)
- A location with specific and relatively stable atmospheric conditions (temperature and humidity) where animals overwinter. These are often sites where a species will congregate.
- Hibernation
- A state of inactivity and greatly reduced metabolism which occurs seasonally for a species that regulates its body temperature primarily through its own bodily functions, rather than by ambient temperature. Hibernation is characterized by low body temperature, slower breathing, reduced heart rate, and low metabolic activity.
- Historical (element occurrence)
- An area of land or water where a species was present historically (not observed in the last 20 to 40 years, NatureServe 2015). In this Recovery Strategy, Historical refers to dates prior to 1999.
- Karst
- A landscape formed in areas of soluble rock such as limestone, dolostone or gypsum. It is characterized by underground drainage that results in features such as sinkholes, caves, and disappearing streams.
- Species at Risk Act (SARA)
- The federal legislation that provides protection to species at risk in Canada. This act establishes Schedule 1 as the legal list of wildlife species at risk. Schedules 2 and 3 contain lists of species that at the time the Act came into force needed to be reassessed. After species on Schedule 2 and 3 are reassessed and found to be at risk, they undergo the SARA listing process to be included in Schedule 1.
- Species at Risk in Ontario (SARO) List
- The regulation made under section 7 of the Endangered Species Act, 2007 that provides the official status classification of species at risk in Ontario. This list was first published in 2004 as a policy and became a regulation in 2008.
- Swarming
- The activity in which male and female bats gather to mate and prepare for hibernation. Swarming usually occurs at the entrance to a hibernaculum.
- White-nose syndrome (WNS)
- A disease of hibernating bats caused by the fungus Pseudogymnoascus destructans (Pd) that has caused widespread mortality of cave-hibernating bats throughout eastern North America, where it was first discovered in New York State in 2006. Signs of white-nose syndrome include a white substance on the muzzle, ears, and wings of an infected bat during hibernation. Mortality from white-nose syndrome is thought to be a result of wing lesions leading to dehydration that causes excessive arousal and depleted fat reserves, which can subsequently cause emaciation and death.
List of abbreviations
ALT: Treed Alvar (southern Ontario ELC, Lee, et al. 1998)
BO: Bog (southern Ontario ELC, Lee, et al. 1998)
COSEWIC: Committee on the Status of Endangered Wildlife in Canada
COSSARO: Committee on the Status of Species at Risk in Ontario
CUM: Cultural Meadow (southern Ontario ELC, Lee, et al. 1998)
CUS: Cultural Savannah (southern Ontario ELC, Lee, et al. 1998)
CUT: Cultural Thicket (southern Ontario ELC, Lee, et al. 1998)
CUW: Cultural Woodland (southern Ontario ELC, Lee, et al. 1998)
ECCC: Environment and Climate Change Canada
ELC: Ecological Land Classification
ESA: Ontario’s Endangered Species Act, 2007
FE: Fen (southern Ontario ELC, Lee, et al. 1998)
FO: Forest (southern Ontario ELC, Lee, et al. 1998)
FOC3: Fresh-Moist Hemlock Coniferous Forest (southern Ontario ELC, Lee, et al. 1998)
FOC4: Fresh-Moist White Cedar Coniferous Forest (southern Ontario ELC, Lee, et al. 1998)
FOD6: Fresh-Moist Sugar Maple Deciduous Forest (southern Ontario ELC, Lee, et al. 1998)
FOD7: Fresh-Moist Lowland Deciduous Forest (southern Ontario ELC, Lee, et al. 1998)
FOD8: Fresh-Moist Poplar-Sassafras Deciduous Forest (southern Ontario ELC, Lee, et al. 1998)
FOM6: Fresh-Moist Hemlock Mixed Forest (southern Ontario ELC, Lee, et al. 1998)
FOM7: Fresh-Moist White Cedar-Hardwood Mixed Forest (southern Ontario ELC, Lee, et al. 1998)
FOM8: Fresh-Moist Poplar-White Birch Mixed Forest (southern Ontario ELC, Lee, et al. 1998)
ISBN: International Standard Book Number
MA: Marsh (southern Ontario ELC, Lee, et al. 1998)
MECP: Ministry of the Environment, Conservation and Parks
MNRF: Ministry of Natural Resources and Forestry
NHIC: Natural Heritage Information Centre
NABat: North American Bat Monitoring Protocol (Loeb et al. 2015)
OA: Open Water (southern Ontario ELC, Lee, et al. 1998)
RBT: Treed Rock Barren (southern Ontario ELC, Lee, et al. 1998)
SA: Shallow Water (southern Ontario ELC, Lee, et al. 1998)
SARA: Canada's Species at Risk Act
SARO List: Species at Risk in Ontario List
SBT: Treed Sand Barren (southern Ontario ELC, Lee, et al. 1998)
SD: Standard Deviation
SE: Standard Error
SW: Swamp (southern Ontario ELC, Lee, et al. 1998)
SWC: Coniferous Swamp (southern Ontario ELC, Lee, et al. 1998)
SWD: Deciduous Swamp (southern Ontario ELC, Lee, et al. 1998)
SWM: Mixed Swamp (southern Ontario ELC, Lee, et al. 1998))
TAT: Treed Talus (southern Ontario ELC, Lee, et al. 1998)
TPW: Tallgrass Woodland (southern Ontario ELC, Lee, et al. 1998)
UTM: Universal Transverse Mercator (system)
References
Banton, Erin, John Johnson, Harold Lee, Gerry Racey, Peter Uhlig, and Monique Wester. 2009. Ecosites of Ontario, Operational Draft. Ecological Land Classification Working Group.
Broders, Hugh G, Graham J Forbes, Stephen Woodley, and Ian D Thompson. 2006. "Range Extent and Stand Selection for Roosting and Foraging in Forest-Dwelling Northern Long-eared Bats and Little Brown Bats in the Greater Fundy Ecosystem, New Brunswick." The Journal of Wildlife Management 70 (5): 1174-1184.
Brown, Jennifer A, Donald F McAlpine, and Rosemary Curley. 2007. "Northern Long-eared Bat, Myotis septentrionalis (Chiroptera: Vespertilionidae), on Prince Edward Island: First Records of Occurrence and Over-wintering." The Canadian Field-Naturalist 121: 208-209.
Browning, Mark H R, and Jeff Bowman. 2017. "An Approach for the Broad-scale Monitoring of Bat Populations in Ontario (Abstract)." 4th North American Congress for Conservation Biology. Toronto, Ontario. 123pp. Accessed March 1, 2019. https://www.xcdsystem.com/scbna/program/3Rny03X/index.cfm?pgid=1315.
Caceres, M Carolina, and Robert M R Barclay. 2000. Myotis septentrionalis.; Mammalian Species 634: 1-4.
Davis, Wayne H, and Russel E Mumford. 1962. "Ecological Notes on the Bat Pipistrellus Subflavus. The American Midland Naturalist 68 (2): 394-398.
Dowling, Zara, Danielle O'Dell, and Karen Beattie. 2017. "The Northern Long-eared Bat, and Other Chiroptera, on Nantucket." Annual Meeting of the Northeast Bat Working Group. Amherst, Massachusetts: Northeast Bat Working Group, January 12.
Environment and Climate Change Canada. 2018. Recovery Strategy for the Little Brown Myotis (Myotis lucifugus), the Northern Myotis (Myotis septentrionalis), and the Tri-colored Bat (Perimyotis subflavus) in Canada. Species at Risk Act Recovery Strategy Series, Ottawa: Environment and Climate Change Canada, ix + 172.
Ethier, Kevin, and Lenore Fahrig. 2011. "Positive Effects of Forest Fragmentation, Independent of Forest Amount, on Bat Abundance in Eastern Ontario, Canada." Landscape Ecology 26: 865-876.
Fenton, M Brock. 1969. "Summer Activity of Myotis lucifugus (Chiroptera: Vespertilionidae) at Hibernacula in Ontario and Quebec." Canadian Journal of Zoology 47: 597-602.
Fenton, M Brock, and Robert M R Barclay. 1980. Myotis lucifugus. Mammalian Species 142: 1-8.
Foster, Rodney W, and Allen Kurta. 1999. "Roosting Ecology of the Northern Bat (Myotis septentrionalis) and Comparisons with the Endangered Indiana Bat (Myotis sodalis). American Society of Mammalogists 80 (2): 659-672.
Fujita, Marty S, and Thomas H Kunz. 1984. "Pipistrellus subflavus." Mammalian Species 228: 1-6.
Heaven, Paul. 2018. County of Haliburton Bats at Risk Assessment: Final Report. Project 17012. Glenside Ecological Services Limited, 81.
Henderson, Lynne, and Hugh G Broders. 2008. "Movements and Resource Selection of the Northern Long-eared Myotis (Myotis septentrionalis) in a Forest-Agriculture Landscape." Journal of Mammalogy 89 (4): 952-963.
Henry, Mickaël, Donald W Thomas, Réal Vaudry, and Michel Carrier. 2002. "Foraging Distances and Home Range of Pregnant and Lactating Little Brown Bats (Myotis lucifugus)." Journal of Mammalogy 83 (3): 767-774.
Jantzen, M K, and M B Fenton. 2013. "The Depth of Edge Influence Among Insectivorous Bats at Forest-Field Interfaces." Canadian Journal of Zoology 91 (5): 287-292.
Layng, Amanda M, Amanda M Adams, Derek E Goertz, Kyle W Morrison, Bruce A Pond, and R Dean Phoenix. 2019. "Bat Species Distribution and Habitat Associations in Northern Ontario, Canada." Journal of Mammalogy 100 (1): 249-260.
Lee, H T, W D Bakowsky, J Riley, J Bowles, M Puddister, P Uhlig, and S McMurray. 1998. Ecological Land Classification for Southern Ontario: First Approximation and its Application. SCSS Field Guide FG-02, Ontario Ministry of Natural Resources, Southcentral Science Section, Science Development and Transfer Branch.
Loeb, Susan C, Thomas J Rodhouse, Laura E Ellison, Cori L Lausen, Jonathan D Reichard, Kathryn M Irvine, Thomas E Ingersoll, et al. 2015. A Plan for the North American Bat Monitoring Program (NABat). General Technical Report SRS-208, Asheville, North Carolina: United States Department of Agriculture Forest Service Research & Development.
Lowe, Amanda J. 2012. Swarming Behaviour and Fall Roost-Use of Little Brown (Myotis lucifugus), and Northern Long-eared Bats (Myotis septentrionalis) in Nova Scotia, Canada. Halifax, Nova Scotia: M.Sc. Thesis, Saint Mary's University.
Owen, Sheldon F, Michael A Menzel, W Mark Ford, Brian R Chapman, Karl V MIller, John W Edwards, and Petra B Wood. 2003. "Home-range Size and Habitat Used by the Northern Myotis (Myotis septentrionalis)." The American Midland Naturalist 150: 352-359.
Perry, Roger W, and Ronald E Thill. 2007. "Tree Roosting by Male and Female Eastern Pipistrelles in a Forested Landscape." Journal of Mammalogy 88 (4): 974-981.
Poissant, Joseph A, Hugh G Broders, and Greg M Quinn. 2010. "Use of Lichen as a Roosting Substrate by Perimyotis subflavus, the Tricolored Bat, in Nova Scotia." Écoscience 17 (4): 372-378.
Quinn, Greg M, and Hugh G Broders. 2007. Roosting and foraging ecology of eastern pipistrelle (Perimyotis subflavus) bats in SW Nova Scotia. Prepared for Nova Scotia Habitat Conservation Fund, c/o Nova Scotia Department of Natural Resources, St. Mary's, Nova Scotia: Department of Biology, Saint Mary's University.
Randall, Lea A, Thomas S Jung, and Robert M R Barclay. 2014. "Roost-site Selection and Movements of Little Brown Myotis (Myotis lucifugus) in Southwestern Yukon." Northwestern Naturalist 95: 312-317.
Schaefer, Katherine. 2017. Habitat Usage of Tri-colored Bats (Perimyotis subflavus) in Western Kentucky and Tennessee Post-White Nose Syndrome. Murray, Kentucky: M.Sc. Thesis, Murray State University (Murray State Digital Commons 33).
Thalken, Marissa M, Michael J Lacki, and Jian Yang. 2018. "Landscape-scale Distribution of Tree Roosts of the Northern Long-eared Bat in Mammoth Cave National Park, USA." Landscape Ecology 33: 1103-1115.
U.S. Fish and Wildlife Service. 2013. 12-month Finding on a Petition to List the Eastern Small-footed Bat and the Northern Long-eared Bat as Endangered or Threatened Species; Listing the Northern Long-eared Bat as an Endangered Species. Federal Register 78(191):61046-61080, United States Fish and Wildlife Service.
—. 2018. "White-nose syndrome occurrence map - by year (2019)." White-nose Syndrome Occurrence by County or District (or portions thereof). October 2. Accessed March 1, 2019. https://www.whitenosesyndrome.org/spreadmap.
van Zyll de Jong, C G. 1985. Handbook of Canadian Mammals. Volume 2: Bats. Ottawa: National Museum of Natural Sciences.
Veilleux, Jacques Pierre, John O Whitaker Jr, and Sherry L Veilleux. 2003. "Tree-Roosting Ecology of Reproductive Female Eastern Pipistrelles, Pipistrellus subflavus, in Indiana." Journal of Mammalogy 84 (3): 1068-1075.
Whitaker, John O. 1998. "Life History and Roost Switching in Six Summer Colonies of Eastern Pipistrelles in Buildings." Journal of Mammalogy 79 (2): 651-659.
Yates, D E, S E Angelo, M W Goodale, and D C Evers. 2011. Bat Mercury Study Examining Footprint Area and Downstream: South River, Virginia - 2009. Report BRI 2009-10. Submitted to DuPont Corporate Remediation Group (Newark, Delaware), and the U.S. Fish and Wildlife Service (Gloucester, Virginia), Gorham, Maine: Biodiversity Research Institute, 57pp.
Personal communications
Browning, Mark. 2019. Discussion and reference to presentation on NABat acoustic monitoring made at the 4th North American Congress for Conservation Biology. Email correspondence to C. Humphrey and H. Fotherby. March 1, 2019. Research Biologist, Ontario Ministry of Natural Resources and Forestry, Wildlife Research & Development Branch, Peterborough, Ontario.
Browning, Mark. 2019. Discussion of Little Brown Myotis maternity colonies recently surveyed, recapture records, and recent hibernacula surveys. Email correspondence to C. Humphrey. March 14, 2019. Research Biologist, Ontario Ministry of Natural Resources and Forestry, Wildlife Research & Development Branch, Peterborough, Ontario.
Davy, Christina. 2019. Confirmation of band returns of Tri-colored Bat from sites surveyed during swarming as well as hibernation in Ontario. Comments received through addendum preparation. September 13, 2019. Research Scientist / Conservation Biologist, SAR, Ontario Ministry of Natural Resources and Forestry, Wildlife Research & Monitoring Section, Peterborough, Ontario.
Entwistle, Cathy. 2019. Confirmation on the location of an observation of Little Brown Myotis in the NHIC observation data. Email correspondence to C. Humphrey. March 7, 2019. Park Interpreter, Ontario Parks.
Holt, Ryan. 2019. Data on roost trees used by Little Brown Myotis in Renfrew County in 2019. Email correspondence to C. Humphrey. September 26 and 29, 2019. M.Sc. candidate, Environmental and Life Sciences, Trent University.
Hooton, Lauren. 2019. Discussion of a Little Brown Myotis maternity colony utilizing an artificial roost structure and a barn in southern Ontario. Email correspondence to C. Humphrey and H. Fotherby. May 13 and May 14, 2019. PhD candidate, Environmental and Life Sciences, Trent University.
Morbey, Yolanda. 2019. Raw data and summary report for Little Brown Myotis and Northern Myotis tracked on the Bruce Peninsula by Jonasson, Guglielmo and Morbey. Email correspondence to C. Humphrey. 2019. Associate Professor, Department of Biology, Western University.
Morningstar, Derek. 2019. Discussion on observations of roosts and hibernating Little Brown Myotis, Northern Myotis, and Tri-colored Bat at various sites in Ontario. Email correspondence to C. Humphrey. March 11, 2019. Ecologist, Myotistar and LGL Limited, Cambridge, Ontario.
Morris, Ed. 2019. Discussion of extant and historic Little Brown Myotis maternity colonies in Northeast Zone Ontario Parks. Email correspondence to C. Humphrey. March 11, 2019. Ecologist, Northeast Zone, Ontario Parks, Sudbury, Ontario.
Schulte-Hostedde, Bridget. 2019. Occurrences of Species at Risk bats and studies undertaken at four Ontario Parks. Email correspondence to C. Humphrey and H. Fotherby. March 4, 2019. Manager, Northeast Zone, Ontario Parks, Sudbury, Ontario.
Thorne, Toby and Eryk Matczak. 2019. Discussion of the Toronto Zoo’s Species at Risk bat survey work undertaken in 2018, including description of roosts used by Little Brown Myotis and Northern Myotis. Telephone conversation with C. Humphrey. March 14, 2019. Native Bat Conservation Program, Toronto Zoo.
Vandermeulen, Josh. 2019. Confirmation on the location of an observation of Tri-colored Bat in the NHIC observation data. Email correspondence to C. Humphrey. March 11, 2019. Biologist, WSP.
Appendix 1. Recovery strategy for the Little Brown Myotis (Myotis lucifugus), the Northern Myotis (Myotis septentrionalis), and the Tri-colored Bat (Perimyotis subflavus) in Canada
Little Brown Myotis (Myotis lucifugus), Northern Myotis (Myotis septentrionalis), and the Tri colored Bat (Perimyotis subflavus): recovery strategy 2018
Official title: Recovery Strategy for the Little Brown Myotis (Myotis lucifugus), the Northern Myotis (Myotis septentrionalis), and the Tri colored Bat (Perimyotis subflavus) in Canada 2018
Species at Risk Act
Recovery strategy series

Northern Myotis, Little Brown Myotis, and Tri-colored Bat
Document information
Environment and Climate Change Canada. 2018. Recovery Strategy for the Little Brown Myotis (Myotis lucifugus), the Northern Myotis (Myotis septentrionalis),and the Tri-colored Bat (Perimyotis subflavus) in Canada. Species at Risk Act Recovery Strategy Series. Environment and Climate Change Canada, Ottawa. ix + 172 pp.
For copies of the recovery strategy, or for additional information on species at risk, including the Committee on the Status of Endangered Wildlife in Canada (COSEWIC) Status Reports, residence descriptions, action plans, and other related recovery documents, please visit the Species at Risk (SAR) Public Registry.
Cover illustration: Left: Northern Myotis, © Jordi Segers; Center: Little Brown Myotis, © Hugh Broders; Right: Tri-colored Bat © Hugh Broders
Également disponible en français sous le titre « Programme de rétablissement de la petite chauve-souris brune (Myotis lucifugus), de la chauve-souris nordique (Myotis septentrionalis) et de la pipistrelle de l’Est (Perimyotis subflavus) au Canada »
© Her Majesty the Queen in Right of Canada, represented by the Minister of Environment and Climate Change, 2018. All rights reserved.
ISBN 978-0-660-28951-9
Catalogue no. En3-4/308-2018E-PDF
Content (excluding the illustrations) may be used without permission, with appropriate credit to the source.
Preface
The federal, provincial, and territorial government signatories under the Accord for the Protection of Species at Risk (1996) agreed to establish complementary legislation and programs that provide for effective protection of species at risk throughout Canada. Under the Species at Risk Act (S.C. 2002, c.29) (SARA), the federal competent ministers are responsible for the preparation of recovery strategies for listed Extirpated, Endangered, and Threatened species and are required to report on progress within five years after the publication of the final document on the SAR Public Registry.
The Minister of Environment and Climate Change and Minister responsible for Parks Canada Agency is the competent minister under SARA for the Little Brown Myotis, Northern Myotis, and Tri-colored Bat and has prepared this recovery strategy, as per section 37 of SARA. To the extent possible, it has been prepared in cooperation with the provinces of British Columbia, Alberta, Saskatchewan, Manitoba, Ontario, Quebec, New Brunswick, Prince Edward Island, Nova Scotia, and Newfoundland and Labrador, as well as the territories of Yukon and Northwest Territories, and the Sahtu Renewable Resources Board, and Wek’èezhìi Renewable Resources Board, as per section 39(1) of SARA.
Success in the recovery of these species depends on the commitment and cooperation of many different constituencies that will be involved in implementing the directions set out in this strategy and will not be achieved by Environment and Climate Change Canada and the Parks Canada Agency, or any other jurisdiction alone. All Canadians are invited to join in supporting and implementing this strategy for the benefit of the Little Brown Myotis, Northern Myotis, and Tri-colored Bat and Canadian society as a whole.
This recovery strategy will be followed by one or more action plans that will provide information on recovery measures to be taken by Environment and Climate Change Canada, the Parks Canada Agency, and other jurisdictions and/or organizations involved in the conservation of the species. Implementation of this strategy is subject to appropriations, priorities, and budgetary constraints of the participating jurisdictions and organizations.
The recovery strategy sets the strategic direction to arrest or reverse the decline of the species, including identification of critical habitat to the extent possible. It provides all Canadians with information to help take action on species conservation. When critical habitat is identified, either in a recovery strategy or an action plan, SARA requires that critical habitat then be protected.
In the case of critical habitat identified for terrestrial species including migratory birds SARA requires that critical habitat identified in a federally protected area
For critical habitat located on other federal lands, the competent minister must either make a statement on existing legal protection or make an order so that the prohibition against destruction of critical habitat applies.
If the critical habitat for a migratory bird is not within a federal protected area and is not on federal land, within the exclusive economic zone or on the continental shelf of Canada, the prohibition against destruction can only apply to those portions of the critical habitat that are habitat to which the Migratory Birds Convention Act, 1994 applies as per SARA ss. 58(5.1) and ss. 58(5.2).
For any part of critical habitat located on non-federal lands, if the competent minister forms the opinion that any portion of critical habitat is not protected by provisions in or measures under SARA or other Acts of Parliament, or the laws of the province or territory, SARA requires that the Minister recommend that the Governor in Council make an order to prohibit destruction of critical habitat. The discretion to protect critical habitat on non-federal lands that is not otherwise protected rests with the Governor in Council.
Acknowledgments
This recovery strategy was prepared by Krista Baker, David Andrews, Kathy St. Laurent (Environment and Climate Change Canada-Canadian Wildlife Service (ECCC-CWS) – Atlantic Region), Ryan Zimmerling, and Sybil Feinman (ECCC-CWS – National Capital Region).
Andrew Boyne (ECCC-CWS – Atlantic Region), Robert McLean, Manon Dubé, Véronique Lalande, Paul Johanson, Véronique Brondex, Marie-Andrée Carrière, Julie Nadeau, Jean-François Déry, Charles Francis, Carolyn Seburn, Veronica Aponte, Marc-André Cyr (ECCC-CWS – National Capital Region), Diana Ghikas, Donna Bigelow, Lynne Burns, Amy Ganton, Dawn Andrews (ECCC-CWS – Prairie Northern Region), Syd Cannings, Tanya Luszcz, Megan Harrison, Darcy Henderson (ECCC-CWS – Pacific Yukon Region), Sylvain Giguère, Pierre-André Bernier (ECCC-CWS – Quebec Region), Krista Holmes, Judith Girard, Marie-Claude Archambault (ECCC-CWS – Ontario Region), Hugh Broders (Saint Mary`s University), Craig Willis (University of Winnipeg), Jordi Segers (Canadian Wildlife Health Cooperative), Derek Morningstar (Myotistar / Golder Associates), Cori Lausen (Wildlife Conservation Society Canada), Mark Brigham (University of Regina), Juliet Craig (British Columbia Community Bat Project Network), Laura Kaupas (University of Calgary), Thomas Jung (Yukon Department of Environment), Joanna Wilson, Ashley McLaren, Stephanie Behrens (Government of Northwest Territories), Purnima Govindarajulu (British Columbia Ministry of Environment), Lisa Wilkinson (Alberta Environment and Parks), Daphne Murphy (Saskatchewan Ministry of Environment), Jay Fitzsimmons, Lesley Hale, Chris Risley, Brian Naylor, Jim Saunders, Vivian Brownell, Katie Paroschy, Sue Russell, Aileen Wheeldon, Todd Copeland, Peter Davis, Chuck McCridden, Megan Rasmussen, Valerie Vaillancourt, Laura Darbey, Bill Greaves, Christopher Martin, April Mitchell (Ontario Ministry of Natural Resources and Forestry), Nathalie Desrosiers, Ariane Massé, Anouk Simard, Isabelle Gauthier (Ministère des Fôrets, de la Faune et des Parcs [Quebec]), Mary Sabine, Maureen Toner (New Brunswick Department of Natural Resources), Garry Gregory (Prince Edward Island Department of Communities, Land, and Environment), Mark Elderkin (Nova Scotia Department of Natural Resources), Shelley Pardy, Bruce Rodrigues, Allysia Park, Sara McCarthy (Newfoundland and Labrador Department of Environment and Conservation), Marie-Josée Laberge, Pippa Shepherd (Parks Canada Agency), and staff at the Department of National Defence provided expertise, document reviews, and/or data during the development of this recovery strategy.
Executive Summary
Little Brown Myotis (Myotis lucifugus), Northern Myotis (Myotis septentrionalis), and Tri-colored Bat (Perimyotis subflavus) are small, insectivorous species of the Family Vespertilionidae. The three species were emergency listed as Endangered on Schedule 1 of the federal Species at Risk Act (SARA) in 2014 because of sudden and dramatic declines across the eastern portions of the ranges of Little Brown Myotis and Northern Myotis, and throughout the entire Canadian range of Tri-colored Bat. These declines are the direct result of white-nose syndrome (WNS).
Little Brown Myotis and Northern Myotis have been confirmed in every province and territory except Nunavut. Approximately 50% and 40% of their global ranges occur in Canada, respectively. Tri-colored Bat has been recorded in Ontario, Quebec, New Brunswick, and Nova Scotia, and 10% of its global population is estimated to occur in Canada.
The primary threat to Little Brown Myotis, Northern Myotis, and Tri-colored Bat is WNS. In areas already affected by WNS, the significance of threats that result in additive mortality to the three species of bats is heightened because the mortality of a small number of the remaining individuals (particularly juveniles and adults) has the ability to impact the survival of local populations, their recovery, and, perhaps, the development of resistance to the fungus that causes WNS. Threats other than WNS include residential and commercial development, energy production and mining, biological resource use, human intrusions and disturbance, natural system modifications and pollution. Potential threats with unknown impacts for all three species include are related to climate change and severe weather.
The feasibility of recovery for Little Brown Myotis, Northern Myotis, and Tri-colored Bat in Canada is unknown. In keeping with the precautionary principle, this recovery strategy has been prepared as per section 41(1) of SARA.
Within WNS-affected areas, the short-term (12-18 years) population objective for both Little Brown Myotis and Northern Myotis is to stop the declining population trends, or if feasible, achieve increasing population trends. Within WNS-affected areas, the long-term (many generations) population objective is a self-sustaining, resilient, redundant and representative population (see section 5: Population and Distribution Objectives for further details and definitions). Within areas not yet known to be affected by WNS, the population objective is to maintain stable population trends, or if feasible, achieve increasing population trends. The distribution objective for both the Little Brown Myotis and Northern Myotis is to maintain (or where applicable restore to) the pre-WNS extent of occurrence.
The short-term population objective for Tri-colored Bat is to stop the declining population trend, or if feasible, achieve an increasing population trend over the next 10 years. The long-term population objective is a self-sustaining, resilient, redundant and representative population. The distribution objective is to restore (then maintain) the pre-WNS extent of occurrence.
Broad strategies aimed at supporting the survival and recovery of Little Brown Myotis, Northern Myotis, and Tri-colored Bat are presented in section 6.2: Strategic Direction for Recovery. Approaches required to meet population and distribution objectives will be different in areas where WNS has already caused dramatic declines, compared to areas not yet affected by WNS.
Critical habitat for these three species is partially identified in this recovery strategy. A schedule of studies is included to obtain the information needed to complete the identification of critical habitat.
One or more action plans for Little Brown Myotis, Northern Myotis, and Tri-colored Bat will be posted on the Species at Risk Public Registry within three years following the final posting of this recovery strategy.
Recovery Feasibility Summary
Based on the following four criteria that Environment and Climate Change Canada uses to establish recovery feasibility, there are unknowns regarding the feasibility of recovery of the Little Brown Myotis, Northern Myotis, and Tri-colored Bat. In keeping with the precautionary principle, a recovery strategy has been prepared as per section 41(1) of SARA, as would be done when recovery is determined to be technically and biologically feasible. This recovery strategy addresses the unknowns surrounding the feasibility of recovery. Tri-colored Bat’s recovery feasibility is assessed separately because, unlike Little Brown Myotis and Northern Myotis, WNS encompasses Tri-colored Bat’s entire Canadian range.
Little Brown Myotis and Northern Myotis
1. Individuals of the wildlife species that are capable of reproduction are available now or in the foreseeable future to sustain the population or improve its abundance.
Yes. In the provinces and territories where WNS has not been detected, these species are apparently abundant throughout much of their range. At present, it is believed that there are currently adequate numbers of breeding individuals in the Prairies and western Canada to sustain the species in Canada or increase their abundances. The number of individuals remaining in most parts of eastern Canada and the United States (U.S.) is very small. Hence, rescue from outside populations (i.e., the U.S.) is not considered likely. However, there are early indications in some portions of their range that a very small proportion of individuals may survive WNS.
2. Sufficient suitable habitat is available to support the species or could be made available through habitat management or restoration.
Unknown. Sufficient suitable summering habitat (i.e., roosting and foraging habitat) is likely available, and it is possible that more could be made available through management or restoration. However, not all locations are known. Suitable wintering habitat (i.e., hibernacula) that is free of the spores that cause WNS is probably sufficient in the Prairies and western and northern Canada, but has substantially declined in many areas of eastern Canada. Although the habitat characteristics of hibernacula are generally known in eastern Canada, the locations of some hibernacula there remain unknown. In the Prairies, western Canada, and northern Canada, the habitat characteristics and locations of hibernacula are not well understood. Currently, it is not possible to identify available, but currently unoccupied wintering habitat that may be needed for recovery for either of these two species.
3. The primary threats to the species or its habitat (including threats outside Canada) can be avoided or mitigated.
Unknown. The primary threat to these species is WNS which is caused by an invasive fungus (Pseudogymnoascus destructans). Spores that cause WNS are introduced to uninfected hibernacula. Limiting the human-assisted spread of WNS by avoiding activities that can cause the spores to spread to uninfected hibernacula may be possible through education and adherence to handling protocols and beneficial management practices (BMPs). Nevertheless, WNS is predicted to continue to spread across Canada from bat-to-bat contact. It is unknown if natural barriers (e.g., Rocky Mountains) may halt or slow the spread of the disease by bats. No widely tested treatment for WNS exists, but intensive research is currently focused on potential treatment and mitigation measures. New research has found substances from certain bacteria and a yeast that inhibit the growth of P. destructans in the laboratory. This research is still in early stages of development and the effectiveness of its application in the field is unknown.
4. Recovery techniques exist to achieve the population and distribution objectives or can be expected to be developed within a reasonable timeframe.
Unknown. Although WNS has primarily spread through Canada by bats, habitat management and stewardship to prevent (or slow) the human-assisted spread of the spores that cause WNS may be effective at delaying loss of individuals while potential treatments and mitigation measures are researched. Conservation of maternity roosts where bats are known to raise young is likely to aid in the recovery of these species. Appropriately designed and tested habitat enhancement techniques that increase the availability of optimal roosting locations for bats to raise young may additionally aid in the recovery of these species. In addition, limiting access and disturbance to hibernacula can have positive effects on population growth.
Tri-colored Bat
1. Individuals of the wildlife species that are capable of reproduction are available now or in the foreseeable future to sustain the population or improve its abundance.
Unknown. In eastern Canada, where this species and WNS are present, Tri-colored Bat continues to persist at very low population levels. Populations also continue to exist in the U.S., although rescue from outside populations is not considered likely. There are no reliable Canadian estimates of population size. At present, it is believed that there are limited individuals capable of reproduction available to increase population abundances.
2. Sufficient suitable habitat is available to support the species or could be made available through habitat management or restoration.
Unknown. Sufficient suitable summering habitat (i.e., roosting and foraging habitat) is likely available, although not all locations are known. Suitable wintering habitat (i.e., hibernacula) that is free of the fungus that causes WNS may be non-existent because Tri-colored Bat’s entire Canadian range overlaps with the areas affected by WNS. Not all locations of hibernacula are known for this species. It is currently not possible to identify available but currently unoccupied habitat that may be needed for recovery.
3. The primary threats to the species or its habitat (including threats outside Canada) can be avoided or mitigated.
Unknown. The primary threat to these species is WNS an invasive fungus (Pseudogymnoascus destructans). No widely tested treatment for WNS exists, but intensive research is currently focused on potential treatment and mitigation measures. New research has found substances from certain bacterium and yeast that inhibit the growth of P. destructans in the laboratory. This research is still in early stages of development and the effectiveness of its application in the field is unknown.
4. Recovery techniques exist to achieve the population and distribution objectives or can be expected to be developed within a reasonable timeframe.
Unknown. Because it is presumed that WNS encompasses the entire range of Tri-colored Bat in Canada, it will be challenging to conduct the necessary research for the treatment of WNS within a reasonable timeframe for recovery. This is an area of intensive ongoing research.
1. Committee on the Status of Endangered Wildlife in Canada (COSEWIC) species assessment information
Date of Assessment: November 2013
Common Name (population): Little Brown Myotis
Scientific Name: Myotis lucifugus
COSEWIC Status: Endangered
Reason for Designation: Approximately 50% of the global range of this small bat is found in Canada. Sub-populations in the eastern part of the range have been devastated by White-nose Syndrome, a fungal disease caused by an introduced pathogen. This disease was first detected in Canada in 2010, and to date has caused a 94% overall decline in known numbers of hibernating Myotis bats in Nova Scotia, New Brunswick, Ontario, and Quebec. The current range of White-nose Syndrome has been expanding at an average rate of 200-250 kilometres per year. At that rate, the entire Canadian population is likely to be affected within 12 to 18 years. There is no apparent containment of the northward or westward spread of the pathogen, and proper growing conditions for it exist throughout the remaining range.
Canadian Occurrence: Yukon, Northwest Territories, British Columbia, Alberta, Saskatchewan, Manitoba, Ontario, Quebec, New Brunswick, Prince Edward Island, Nova Scotia, Newfoundland and Labrador
COSEWIC Status History: Designated Endangered in an emergency assessment on February 3, 2012. Status re-examined and confirmed in November 2013.
Date of Assessment: November 2013
Common Name (population): Northern Myotis
Scientific Name: Myotis septentrionalis
COSEWIC Status: Endangered
Reason for Designation: Approximately 40% of the global range of this northern bat is in Canada. Sub-populations in the eastern part of the range have been devastated by White-nose Syndrome, a fungal disease caused by an introduced pathogen. This disease was first detected in Canada in 2010 and to date has caused a 94% overall decline in numbers of known hibernating Myotis bats in Nova Scotia, New Brunswick, Ontario, and Quebec hibernacula compared with earlier counts before the disease struck. Models in the northeastern United States for Little Brown Myotis predict a 99% probability of functional extirpation by 2026. Given similar life history characteristics, these results are likely applicable to this species. In addition to its tendency to occur in relatively low abundance levels in hibernacula, there is some indication this species is experiencing greater declines than other species since the onset of White-nose Syndrome. The current range of White-nose Syndrome overlaps with approximately one third of this species' range and is expanding at an average rate of 200 to 250 kilometres per year. At that rate, the entire Canadian population will likely be affected within 12 to 18 years. There is no apparent containment of the northward or westward spread of the pathogen, and proper growing conditions for it exist throughout the remaining range.
Canadian Occurrence: Yukon, Northwest Territories, British Columbia, Alberta, Saskatchewan, Manitoba, Ontario, Quebec, New Brunswick, Prince Edward Island, Nova Scotia, Newfoundland and Labrador
COSEWIC Status History: Designated Endangered in an emergency assessment on February 3, 2012. Status re-examined and confirmed in November 2013.
Date of Assessment: November 2013
Common Name (population): Tri-colored Bat
Scientific Name: Perimyotis subflavus
COSEWIC Status: Endangered
Reason for Designation: This bat is one of the smallest bats in eastern North America. Approximately 10% of its global range is in Canada, and it is considered rare in much of its Canadian range. Declines of more than 75% have occurred in the known hibernating populations in Quebec and New Brunswick due to White-nose Syndrome. This fungal disease, caused by an invasive pathogen, was first detected in Canada in 2010, and has caused similar declines in Little Brown Myotis and Northern Myotis in eastern Canada and the northeastern United States. Most of the Canadian range of the species overlaps with the current White-nose Syndrome range, and further declines are expected as more hibernacula continue to become infected.
Canadian Occurrence: Ontario, Quebec, New Brunswick, Nova Scotia
COSEWIC Status History: Designated Endangered in an emergency assessment on February 3, 2012. Status re-examined and confirmed in November 2013.
2. Species status information
Approximately 50%, 40%, and 10% of the global ranges of Little Brown Myotis, Northern Myotis, and Tri-colored Bat, respectively, occur in Canada (COSEWIC 2013).
Little Brown Myotis, Northern Myotis, and Tri-colored Bat were listed as Endangered in Canada under Schedule 1 of the Species at Risk Act (SARA, c. 29) in November 2014 by an emergency listing order. Little Brown Myotis and Northern Myotis were listed as Endangered in January 2013 on the Species at Risk in Ontario (SARO) List (O. Reg. 230/08) under Ontario’s Endangered Species Act, 2007 (OMNRF 2015). The three species also receive protection under Ontario’s Fish and Wildlife Conservation Act, 1997 as specially protected mammals. All three species were listed as Endangered by the New Brunswick Species at Risk Act in June 2013 and were added to the list of animals protected under the Nova Scotia Endangered Species Act in July 2013. Little Brown Myotis and Northern Myotis were listed as Endangered under Manitoba’s Endangered Species and Ecosystems Act in June 2015.
These species are not listed under the provincial and territorial endangered species legislations of Yukon, British Columbia, Northwest Territories, Alberta, Saskatchewan, Quebec, or Newfoundland and Labrador, although they are afforded some level of protection (individuals and/or habitat) under the Wildlife Acts of these provinces and territories. In Quebec, Tri-colored Bat is listed on the Liste des espèces susceptibles d’être désignées menacées ou vulnérables (list of wildlife species likely to be designated threatened or vulnerable). This list is produced according to the Loi sur les espèces menacées ou vulnérables (RLRQ, c E-12.01) (Act respecting threatened or vulnerable species) (CQLR, c E-12.01), its inclusion on this list could lead to the adoption of some measures beneficial to the species. In Saskatchewan, The Wildlife Amendment Regulation, 2013 removed all Chiroptera from the list of unprotected wildlife, thereby granting them protection from all harm. These species are not listed, nor do they receive protection under the Wildlife Conservation Act in Prince Edward Island. The Province of Newfoundland and Labrador are in the process of assessing the bat species for inclusion on their Endangered Species Act. There are no confirmed records of these species in Nunavut, but there has been limited survey coverage.
NatureServe (2015) ranks for Canada and the United States (U.S.) are presented in Table 1.
Region | Little Brown Myotis | Northern Myotis | Tri-colored Bat | Presence of WNS (winter discovered) |
---|---|---|---|---|
Global | G3G4 | G1G2 | G3G4 | - |
United States | N3 | N1N2 | N3N4 | √ (2006-2007) |
Canada | N3 | N2N3 | N2N3 | - |
Newfoundland | S4 | S2S3 | Not Applicable | - |
Labrador | S4 | SNR | Not Applicable | - |
Nova Scotia | S1 | S2 | S1 | √ (2010-2011) |
PEI | S1 | S1 | Not Applicable | √ (2012-2013) |
Quebec | S1 | S1 | S1 | √ (2009-2010) |
New Brunswick | S1 | S1 | S1 | √ (2010-2011) |
Ontario | S4 | S3 | S3? | √ (2009-2010) |
Manitoba | S2N, S5B | S3S4N, S4B | Not Applicable | - |
Saskatchewan | S5B, S5N | S4B, SNRN | Not Applicable | - |
Alberta | S5 | S2S4 | Not Applicable | - |
British Columbia | S4 | S3S4 | Not Applicable | - |
NT | S2 | S2 | Not Applicable | - |
Yukon | S1S3 | S1S2 | Not Applicable | - |
Types of ranks: G = global conservation status rank, N = national conservation status rank, and S = sub-national (provincial or territorial) ranks.
Definitions of rank: 1 = critically imperiled; 2 = imperiled; 3 = vulnerable; 4 = apparently secure; 5 = secure; SNR = unranked; SU = unrankable; B = breeding; N = non-breeding.
3 Species information
3.1. Species description
Little Brown Myotis
Little Brown Myotis is a small (7-9 g) brown bat with black ears, black wings, and a black tail membrane (van Zyll de Jong 1985). Its wingspan is approximately 22-27 cm. Females tend to be slightly larger than males (Harvey et al. 2011). Compared to other mammals, Little Brown Myotis has a long lifespan; some individuals live more than 30 years (Davis and Hitchcock 1995). When acoustically recorded in treed or otherwise cluttered environments, Little Brown and Northern Myotis produce echolocation calls that are very similar and can be confused with other Myotis species.
Northern Myotis
Northern Myotis, also known as Northern Long-eared Bat, is a small bat (5-8 g) similar in size and colouration to Little Brown Myotis, but is generally distinguishable by its longer ears that extend beyond the nose when pressed forward, longer tail, and larger wing area (Caceres and Barclay 2000, Harvey et al. 2011). It can also be distinguished by its tragus
Tri-colored Bat
Tri-colored Bat, formerly known as Eastern Pipistrelle (Pipistrellus subflavus), has a distinct colouration; each hair is black at the base, yellow in the middle, and brown at the tip giving the bat an overall reddish-brown to yellowish-brown colour (Harvey et al. 2011). Its ears and face are brown, forearms are orange-red or pinkish, and its wings and flight membranes are blackish (Fujita and Kunz 1984, Naughton 2012). Tri-colored Bat is similar in size and weight (5-9 g) to Little Brown Myotis and Northern Myotis (Fujita and Kunz 1984, van Zyll de Jong 1985, Farrow and Broders 2011).
3.2. Species population and distribution
Distribution
Little Brown Myotis
Little Brown Myotis has been confirmed in every province and territory throughout Canada with the exception of Nunavut where no known observations meeting evidentiary standards exist (i.e., recorded but not confirmed) (COSEWIC 2013). In general, its Canadian distribution includes the boreal forest south of the treeline through to the U.S. border (van Zyll de Jong 1985, Grindal et al. 2011, Burles et al. 2014) (Figure 1).
The northern limit of its distribution is difficult to define because of limited survey effort and difficulties related to survey logistics (e.g., large area, few roads – see Jung et al. 2014 for a more comprehensive explanation). Few maternity roosts or hibernacula have been located in the northern portions of the range (COSEWIC 2013); however, Wilson et al. (2014) observed reproductive females and maternity colonies of Little Brown Myotis in southwest and south central Northwest Territories. The species is also found south of 64° in Yukon (Slough and Jung 2008). Hibernacula have been confirmed in the Northwest Territories though no hibernacula have been found in Yukon (Slough and Jung 2008, Wilson et al. 2014). Scattered records from Nunavut and northern Northwest Territories exist (i.e., north of the defined range in Figure 1); however, it is unclear if these records represent resident breeding individuals or extralimital

Long description
Approximate North American distribution (range) map of Little Brown Myotis (Myotis lucifugus) (grey-filled area) and overlap with white-nose syndrome, Pseudogymnoascus destructans, (WNS) (black-diagonal cross-fill) as of May 2018. Recorded, but unconfirmed occurrences of Little Brown Myotis in the Northwest Territories and Nunavut are indicated by question marks (“?”), whereas an extralimital observation (outside defined range as represented by the grey-filled area) is represented as a black dot. There are confirmed occurences of the species in every Canadian province and territory except Nunavut (occurrence unconfirmed).
The species’ continental range is continuous, and its northern limit extends from central Alaska (northernmost point) through north-central Yukon and the southern Northwest Territories along the boreal forest treeline to the northern portions of Saskatchewan, Manitoba, Ontario, Quebec, and Newfoundland and Labrador. The western range limit extends from the Alaskan coastline to the northernmost tip of the Baja California Peninsula into Mexico. The southwestern range limit extends from the Baja California Peninsula along the US-Mexico border into north-central Mexico. The south-central range limit extends from northern Texas into the northern portions of the US Gulf States (Louisiana, Mississipi, and the southern borders of Alabama and Georgia). The eastern range limit extends from northern Newfoundland and Labrador along the Atlantic coastline to southern Georgia in the US. The distribution of white-nose syndrome overlaps the species’ central-eastern range and a small area in Newfoundland.
Northern Myotis
Northern Myotis has been confirmed in every province and territory throughout Canada with the exception of Nunavut (van Zyll de Jong 1985, Brown et al. 2007, Henderson et al. 2009, Park and Broders 2012, Broders et al. 2013, Reimer and Kaupas 2013, CWHC 2018) (Figure 2). Its Canadian distribution includes the boreal forest south of the treeline and into the montane forests of the west and deciduous and mixedwood forests of the east. It is mostly absent from the Canadian Prairies, and when it is found outside of forested regions, it is found in forest remnants or at hibernacula (Turner 1974).
Similar to Little Brown Myotis, the northern limit of Northern Myotis’ range is difficult to determine due to limited survey effort and difficulties related to survey logistics (e.g., large area, few roads). However, Northern Myotis has been confirmed breeding in Yukon (Lausen et al. 2008) and the Northwest Territories (Wilson et al. 2014). Hibernation sites have not been recorded in Yukon (Jung et al. 2006, Slough and Jung 2007), but likely exist in the Northwest Territories (Wilson et al. 2014).

Long description
Approximate North American distribution (range) map of Northern Myotis (Myotis septentrionalis) (grey-filled area) and overlap with white-nose syndrome, Pseudogymnoascus destructans, (WNS) (black-diagonal cross-fill) as of May 2018. Recorded, but unconfirmed occurrence of Northern Myotis in Ontario is indicated by a question mark (“?”). There are confirmed occurrences of the species in every Canadian province and territory except Nunavut (occurrence unconfirmed).
The species’ continental range is near-continuous, and its northern limit extends from southeastern Yukon/southwestern Northwest Territories into the north-central portions of the Canadian Praires and Ontario, Quebec, Newfoundland and Labrador. The southern range covers the south-central US states but the species is largely absent from the US Midwest and central prairies. From west to east, the species’ range extends from the boreal forest of western Canada (British Columbia) across the north-central portions of the Canadian prairie provinces, northern Ontario and north-central Quebec to southeastern Newfoundland. The eastern range limit of the species extends from the interior of central Georgia and northwestern South Carolina in the US, along the Atlantic coastline into southeastern Newfoundland and southeastern Labrador. The species also has a discontinuous range in the southeastern part of the US with isolated occurrences in Alabama, Florida, Georgia and central South Carolina. The distribution of white-nose-syndrome overlaps the eastern portion of the species’ North American range and a small area in Newfoundland.
Tri-colored Bat
The Canadian range of Tri-colored Bat encompasses mainland Nova Scotia, southern New Brunswick, Quebec and Ontario (van Zyll de Jong 1985, Fraser et al. 2012) (Figure 3), although some populations may be isolated within this range (Broders et al. 2003). Breeding records for Tri-colored Bat have been confirmed in Nova Scotia (Broders et al. 2003) though breeding was not observed in New Brunswick (Broders et al. 2001). Although detections of Tri-colored Bat have been recorded from Ontario and Quebec in the summer (COSEWIC 2013), it is unclear if breeding has been confirmed. It has been recorded in hibernacula in all provinces within its range, albeit rarely and/or in relatively small numbers (COSEWIC 2013).

Long description
Approximate North American distribution (range) map of Tri-colored Bat (Perimyotis subflavus) (grey-filled area) and overlap with white-nose syndrome, Pseudogymnoascus destructans, (WNS) (black-diagonal cross-fill) as of May 2018. Recorded, but unconfirmed occurrences of Tri-colored Bat in Ontario and Quebec are indicated by a question mark (“?”), whereas northern range extralimital records are indicated by black dots. The species’ presence in Canada is limited to the central-eastern provinces (Ontario, Quebec, southern New Brunswick and Nova Scotia).
The species' continental range is continuous, but confined to the central and eastern portions of the US and Canada; its northern limit extends along the Great Lakes and the Laurentians in Canada. The species’ range narrows/contracts from the southern US Gulf States into Mexico, where its southern limit extends along the western Gulf of Mexico coastline as far south as Guatemala. The distribution of white-nose syndrome overlaps the northeastern portion of the species’ range and a small area in Newfoundland.
Population
This recovery strategy defines two periods relevant to the discussion of bat populations in Canada: the period prior to the arrival of WNS in Canada (up to and including 2009) and the period after the arrival of WNS in Canada (2010 and onwards). However, it should be noted that the population sizes and the relative abundance of the three bat species in Canada are unknown pre- and post-WNS (both in Canada and in North America) and, therefore, it is challenging to obtain accurate estimates of abundance and consequently species-specific declines in the Canadian populations.
In the northeastern U.S., Langwig et al. (2012) estimated that bat populations (all species) in general, prior to the arrival of WNS, were growing at an average rate of 8% per year. Population trend analyses of hibernacula data from across the U.S. indicated the population trends of these three species of bat specifically were relatively stable (i.e., a positive or negative trend was unable to be detected) (Ellison et al. 2003, Frick et al. 2010a, Frick et al. 2010b). Count data at hibernacula during the winter are often used to determine relative abundance and infer population trends. Substantial intra- and inter-annual variation in the number of hibernating bats (and species) can exist within a hibernaculum (Trombulak et al. 2001); nonetheless, winter hibernacula data are likely an accurate reflection of the population status in all three bat species’ populations (COSEWIC 2013). In addition, summer survey data corroborate observations collected at hibernacula (COSEWIC 2013, Natureserve 2015).
Since the arrival of WNS to North America in 2006, the most precipitous declines in North American wildlife in recorded history have been observed (Kunz and Tuttle 2009). An estimated one million bats (multiple species) died in the northeastern U.S. within three years of the arrival of WNS (Kunz and Tuttle 2009), and an estimated 5.7 to 6.7 million bats died within six years of its arrival (U.S. Fish and Wildlife Service 2012). In Canada, the total number of Myotis spp. bats recorded in Nova Scotia, New Brunswick, Ontario, and Quebec hibernacula declined by approximately 94% between 2010 and 2012 (COSEWIC 2013). In Quebec, Nova Scotia, and New Brunswick, following the arrival of WNS, some hibernacula no longer have any individuals of these species of bats present (McAlpine and Vanderwolf, unpub. data in COSEWIC 2013, Mainguy and Desrosiers 2011, H. Broders pers. comm. 2015).
There is limited information related to the proportion of bat populations found in eastern Canada versus western Canada. In Canada, 95% of records of hibernating Myotis spp. bats are from Nova Scotia to Manitoba, while relatively few had been recorded west of Manitoba (COSEWIC 2013). Fewer hibernacula have been found in the western provinces and northern territories as compared to the east (excluding Newfoundland and Labrador where few hibernacula are also known); furthermore, hibernacula in the east tended to have more individuals per site (>10,000) compared to hibernacula in the north and west (<1000 per site) (Nagorsen and Brigham 1993, Olson et al. 2011). Some researchers suggest that a large proportion of the Little Brown Myotis population prior to WNS resided in the northeastern United States (Kunz and Reichard 2010). Genetic evidence also exists to suggest populations in the east were larger than populations in the west (Wilder 2014). However, bats in northern and western Canada may not necessarily be less abundant but instead tend to overwinter singly or in small numbers, making it more difficult to obtain accurate population estimates (i.e., many sites with small numbers of bats in the north and west compared to few sites with large numbers in the east). Comparisons between eastern and western population levels should be interpreted with caution because of the survey limitations previously mentioned for the north in addition to issues in the west (i.e., British Columbia) in differentiating Little Brown Myotis from Yuma Myotis (Myotis yumanensis) (COSEWIC 2013).
Little Brown Myotis
Pre-WNS
Evidence from multiple surveys suggests that Little Brown Myotis was probably the most common bat throughout much of Canada, and still is in many areas outside of those impacted by WNS (COSEWIC 2013). Little Brown Myotis is thought to be relatively common in the northern limits of its range, although abundance is difficult to estimate because of previously discussed issues with surveying populations in the north (COSEWIC 2013, Jung et al. 2014).
The Canadian population size of Little Brown Myotis prior to the arrival of WNS is unknown but likely exceeded one million individuals (COSEWIC 2013). Frick et al. (2010a) estimated the population was 6.5 million individuals in the northeastern U.S. as of 2006 which further supports numbers in excess of 1 million for Canada. NatureServe (2015) estimated the global population size to be 100,000 to >1,000,000 individuals prior to WNS. Data from known hibernacula are incomplete but, prior to the arrival of WNS, some known hibernacula were used by thousands to tens of thousands of bats in southern Ontario, Quebec, Nova Scotia, New Brunswick, and Manitoba (Fenton 1970, Scott and Hebda 2004, Mainguy and Desrosiers 2011).
Post-WNS
NatureServe (2015) states that the current global population size is unknown but likely still exceeds 100,000 individuals. Model results predicted that Little Brown Myotis will be functionally extirpated
Northern Myotis
Pre-WNS
Evidence suggests that Northern Myotis is less common than Little Brown Myotis, in part, because they have a more restricted distribution within Canada and are reliant on forested areas (COSEWIC 2013). Observations made during summer indicate that the species is relatively common in the southern Northwest Territories and uncommon at the western and northern edges of their range (Jung et al. 2014, Wilson et al. 2014). At some eastern sites (e.g., Newfoundland and Labrador, Prince Edward Island, and Nova Scotia), numbers were more or less equal to Little Brown Myotis (Park and Broders 2012). However, counts obtained from individual hibernacula in the winter indicate relatively few (i.e., <100) Northern Myotis (Barbour and Davis 1969, Amelon and Burhans 2006). This may be a result of difficulties in detecting Northern Myotis in hibernacula because they are often found in deep cracks (COSEWIC 2013). In addition, it is difficult to distinguish between Little Brown Myotis and Northern Myotis while conducting visual winter hibernacula counts.
The Canadian population size of Northern Myotis is unknown but is believed to have also exceeded one million individuals before the arrival of WNS (COSEWIC 2013). In contrast, NatureServe (2015) estimated that the global population size was relatively small prior to WNS (2,500 to 100,000 individuals). However, NatureServe notes that this estimate is supported by low counts at hibernacula (which may be related to issues with detectability). In addition, the NatureServe estimate appears to be primarily based on counts within the U.S. part of the range. Based on Harvey (1992) and D. Morningstar (pers. comm. 2015), the species is probably more common and abundant in the northern part of its global range (i.e., boreal) than in the south (COSEWIC 2006).
Post-WNS
Declines of Northern Myotis populations in the northeastern U.S. have occurred at the same rate as Little Brown Myotis; an expected result given the two species have similar life history traits and often share same hibernacula. Thus, it was predicted that Northern Myotis will likely also be functionally extirpated (i.e., 1% of pre-WNS population) in the northeastern U.S. by 2026 (Frick et al. 2010a). However, new evidence for Little Brown Myotis suggests that low numbers of individuals are surviving the infection and survival rates have increased at locations previously decimated by WNS, a trend which may also apply to Northern Myotis. The entire Canadian range of Northern Myotis is expected to be impacted by WNS between 2025 and 2028 (COSEWIC 2013). With the 2016 detection of WNS in Washington State, this may occur sooner than anticipated in 2013.
Tri-colored Bat
Pre-WNS
Although too little data exist to reliably estimate the population size of Tri-colored Bat, the species was relatively rare in the Maritimes, Quebec, and in parts of Ontario (COSEWIC 2013). It is also rare in the adjacent states of Vermont (Darling and Smith 2011) and Maine (Zimmerman and Glanz 2000). In addition to being uncommon, Tri-colored Bats tend to hibernate solitarily within hibernacula, often within the deepest parts of the cave where human access is limited, and thus, may be more difficult to detect during hibernation than other species (Hitchcock 1949, Fujita and Kunz 1984, Sandel et al. 2001, COSEWIC 2013).
The Canadian population size of Tri-colored Bat prior to WNS is unknown; however, the COSEWIC (2013) status report provides <20,000 individuals in Canada as a rough estimate. NatureServe (2015) estimated that the global population size was between 10,000 to 1,000,000 individuals prior to WNS. In Nova Scotia, it was estimated that between 1,000 and 2,000 adult females existed (H. Broders, pers. comm. in COSEWIC 2013). Across the Canadian range, the species accounted for between 0.2 to 4.5% of individuals counted at various hibernacula (Hitchcock 1949, 1965, Mainguy and Desrosiers 2011, Vanderwolf et al. 2012).
Post-WNS
The Tri-colored Bat population declines in areas affected by WNS in Canada and the U.S. are likely similar to that observed in Little Brown Myotis and Northern Myotis, though the declines observed in this species are less straightforward (COSEWIC 2013). In part, this is because of the small numbers of Tri-colored Bats that have been recorded from monitored Canadian hibernacula and because the species shows evidence of a seasonal latitudinal migration (Fraser et al. 2012), both of which may affect interpretation of Canadian population trends.
Declines observed since the arrival of WNS have been variable. The average population decline in five northeastern states was 75% (range 16 to 95%) between 2006 and 2010, with 13 of 36 hibernacula declining 100% (Turner et al. 2011). Acoustic monitoring during the summers of 2007-2009 in New York yielded similar population declines to those listed above (i.e., 78% between 2008 and 2009) (Dzal et al. 2011). In eastern Ontario, Quebec, and Nova Scotia there are indications the Tri-colored Bat population may have declined by as much as 94% compared to pre-WNS populations (Mainguy and Desrosiers 2011, L. Hale, pers. comm. in COSEWIC 2013). In southern Ontario, significant declines were also noted at eight locations where acoustic monitoring was performed prior to the arrival of WNS and again in 2014 (D. Morningstar, pers. comm. 2015). In New Brunswick, declines at individual hibernacula have ranged from 30% to more than 75% (D. McAlpine and K. Vanderwolf, unpub. data in COSEWIC 2013, D. McAlpine pers. comm. in COSEWIC 2013). NatureServe (2015) states that the current global population size is unknown but likely still exceeds 10,000 individuals.
3.3 Needs of Little Brown Myotis, Northern Myotis, and Tri-colored Bat
The habitat requirements of temperate-region bats vary by season. The habitat is composed of (1) overwintering habitat (i.e., hibernacula, such as caves, abandoned mines, and wells) used for hibernation and overwinter survival, (2) summering habitat that includes roosting habitat (for maternity roosts and males) and foraging habitat within commuting range of the roosts (Sasse and Perkins 1996, Norquay et al. 2013), and (3) swarming habitat used in the late summer and early fall for mating and socializing (Fenton 1969, Randall and Broders 2014). Swarming sites are also typically used as hibernacula (Fenton 1969, Randall and Broders 2014).
Overwintering and swarming habitat
Hibernating bats survive the winter using stored fat reserves accumulated during the summer and autumn (Jonasson and Willis 2011). Hibernation allows year-round resident, insect-eating bats to persist in a region when ambient temperature declines and insects are not available in winter. Though winter in Canada is commonly understood as the months of December, January and February, the period that bats hibernate may extend longer in some locations (e.g., in the north). Hibernating bats minimize use of fat reserves by decreasing metabolic rate and body temperature to within a few degrees of the ambient temperature in the hibernaculum (i.e., they enter torporTorpor is a state of physical inactivity (reduced body temperature and metabolic rate).) (Henshaw and Folk 1966).
Hibernacula for Little Brown Myotis, Northern Myotis, and Tri-colored Bat are generally underground openings, including caves, abandoned mines, wells, and tunnels, but at some sites only specific sections of the site will be used for hibernation. The sections used for hibernating typically have a temperature range between 2˚C and 10˚C (Fenton 1970, Anderson and Robert 1971, Vanderwolf et al. 2012), and relative humidity levels > 80% to reduce evaporative water loss (Cryan et al. 2010, but see Kurta 2014). Structural features, such as number of openings, cave size and length, and angle of chambers can influence the stability and levels of humidity and temperature (Davis 1970, Raesly and Gates 1987). Because of the specific, stable microclimates required by bats, hibernacula are typically used year after year by overwintering bats.
Stable microclimates are preferred by bats because temperature fluctuations can cause arousals from torpor. Bats will arouse from torpor to access water, groom, and mate (Whitaker and Rissler 1993, Thomas 1995), but they consume a significant portion of their limited energy reserve during arousals (Thomas et al. 1990). Relocating to more suitable sites can accelerate the depletion of limited energy reserves, but may also be used as an adaptation for long-term energy conservation.
Where their distributional ranges overlap, all three bat species may occur in the same hibernaculum, but may be found in different sections. Northern Myotis and Tri-colored Bat do not typically overwinter in buildings, but Little Brown Myotis may overwinter in buildings in western Canada (C. Lausen, pers. comm. 2015), where winter temperatures are relatively high. Little is known regarding the overwintering habitat of Little Brown Myotis and Northern Myotis in western Canada.
Bats congregate in swarming habitat in the late summer and early fall. Swarming sites may function as mating sites, stopover locations during migration, social sites for information transfer, and/or allow individuals to assess potential sites for overwintering (Fenton 1969, Randall and Broders 2014). Swarming behaviour often occurs in and around entrances or openings of hibernacula. Both Little Brown Myotis and Northern Myotis often swarm and hibernate at the same locations (Randall and Broders 2014), but the proportion of bats that visit a swarming site in relation to those that stay at the site for hibernation is unknown (Johnson et al. 2015), and possibly quite low in some cases (M. Davis, pers. comm. 2016). Swarming groups of Little Brown Myotis and Northern Myotis in eastern Canada are comprised of individuals from various summering sites and therefore gene pools may span relatively large areas (Burns et al. 2014, Johnson et al. 2015, Segers and Broders 2015).
Few studies have attempted to characterize external habitat features that predict selection of hibernacula and swarming sites by bats. In Nova Scotia, a survey of natural and anthropogenic swarming sites (abandoned mines), also assumed to be hibernacula, revealed that the amount of entrance shelter (i.e., canopy cover and/or rock faces that provide protection from weather elements), watercourse length within a 2 km radius of the site, and total chamber length of the hibernaculum were significant predictors of Little Brown Myotis and Northern Myotis swarming activity (Randall and Broders 2014). Generally, swarming sites had more exposed entrances, greater total length of rivers, creeks, and streams within a 2 km radius, and deeper / longer chamber lengths, than sites surveyed where swarming activity levels were low or absent (Randall and Broders 2014). For example, an 10% increase in the degree of entrance shelter resulted the probability of swarming declining by 50% and the probability of swarming doubled with an increase of 10 km of stream length within a 2 km radius (Randall and Broders 2014).
Little Brown Myotis
Little Brown Myotis appear to derive energetic and water conservation benefits from clustering while hibernating. In Michigan, Kurta and Smith (2014) found that 78% of individuals hibernated in clusters and were more likely to be found in clusters (rather than solitarily) at lower temperatures. In western Canada, the number of Little Brown Myotis hibernating together may be substantially less than in northeastern North America, although clusters as large as 52 individuals have been observed in a hibernaculum containing 3000 individuals in southwestern Northwest Territories (Lausen 2011); bats likely hibernate singly or in small groups west of the Rocky Mountains (Jung et al. 2014). A recent radio-telemetry study in the temperate rainforest of southeast Alaska found Little Brown Myotis hibernating solitarily in rock scree on steep, forested hillsides and beneath root wads of trees and stumps (K. Blejwas, pers. comm. 2015). Little Brown Myotis exhibit high fidelity to hibernacula (Norquay et al. 2013). A mark-recapture study in Manitoba and northwestern Ontario found only 4% of marked individuals relocated to an alternate hibernaculum within the study period (Norquay et al. 2013).
Northern Myotis
Northern Myotis may hibernate in cooler sections of a cave, compared to Little Brown Myotis (Barbour and Davis 1969). In a study of abandoned mines in northern Michigan, Northern Myotis and Little Brown Myotis co-occurred in 92% of the mines occupied by Myotis spp., but 75% of Northern Myotis individuals hibernated alone (Kurta and Smith 2014). Northern Myotis will generally return to the same hibernaculum, but not always in consecutive years (Caceres and Barclay 2000). Naughton (2012) noted that “they are loyal to a group of hibernacula rather than a single one”.
Tri-colored Bat
Tri-colored Bat is considered to have the most rigid overwintering habitat requirements of the three species. They often select the deepest part of caves or mines where temperature is the least variable, have strong humidity level preferences, and use warmer walls than other species (Fujita and Kunz 1984, Raesly and Gates 1987, Briggler and Prather 2003, Kurta and Smith 2014). A study of hibernacula in New Brunswick noted Tri-colored Bats hibernating low on the cave walls (Vanderwolf et al. 2012). Although Tri-colored Bats tend to use the same hibernacula as Little Brown Myotis and Northern Myotis, relatively few (i.e., ≤10) Tri-colored Bats have been recorded within any one hibernacula in Canada, possibly because they tend to hibernate solitarily (i.e., not in clusters) in the deepest sections of the caves/mines. Tri-colored Bats exhibit high fidelity to hibernacula (Sandel et al. 2001, Damm and Geluso 2008).
Summering habitat
Roosting habitat
Roosts provide thermal regulation, shelter from weather and predation, and can be sites for social interaction (Kunz 1982, Barclay and Kurta 2007). Individuals may switch roosts regularly and therefore, may use a network of roosts in a roosting area (Barclay and Brigham 1996, Sasse and Perkins 1996, Caceres and Barclay 2000). The tendency to switch roosts may depend on species, sex, age, reproductive status, and roost type (e.g., natural or anthropogenic) (Garroway and Broders 2008, Randall et al. 2014).
Roost selection is a function of numerous characteristics occurring at a range of spatial scales (Fabianek et al. 2011). For example, at the scale of the roosting structure, tree species, diameter, height, stage of decay, availability of roosting medium, sun exposure, and other characteristics may affect roost selection (Garroway and Broders 2008, Slough 2009, Poissant et al. 2010, Olson and Barclay 2013). At the stand scale, roost selection may be a function of canopy gaps, number of available snags, tree density, proximity to water, etc. (Kalcounis-Rüppell et al. 2005, Garroway and Broders 2008, Henderson and Broders 2008). At the landscape scale, characteristics such as forest age, composition, and degree of fragmentation may affect roost selection (Henderson and Broders 2008, Fabianek et al. 2011). The species may also use treed and forested habitat in urban and suburban areas for roosting, in addition to man-made structures found within urban and suburban landscapes (Little Brown Myotis, in particular).
Many bat species (including Little Brown Myotis, Northern Myotis, and Tri-colored Bat) preferentially roost in older forest stands, compared to young forests (Barclay and Brigham 1996). Older forests likely provide increased snag availability for roosting (Crampton and Barclay 1996, Krusic et al. 1996) and foraging habitat under a relatively closed canopy (Jung et al. 1999).
Females generally give birth and raise pups in maternity colonies in the spring/summer. Because of roost switching behavior, a colony can be defined as an assemblage of roosting groups comprised of individuals that regularly associate and groups that intermix (Olson and Barclay 2013). Roosting in groups likely aids social thermoregulation and energy savings (Willis and Brigham 2007).
Males of all three species roost during the daytime in a variety of structures, and often switch sites during the summer. Male roosting habitat includes rock crevices, raised bark, foliage, and tree cavities (Fenton and Barclay 1980b, Caceres and Barclay 2000, Broders and Forbes 2004, Huynh 2009, Randall et al. 2014, Fabianek et al. 2015). Male Little Brown Myotis and Northern Myotis often roost in tall snags with large diameters in the early to middle stages of decay and located in or near small open patches within mature to over mature forest (Broders and Forbes 2004, Jung et al. 2004, Fabianek et al. 2015).
Little Brown Myotis
Little Brown Myotis is one of the few bat species that uses buildings and other anthropogenic structures (e.g., bat boxes, bridges, and barns) to roost (particularly for maternity roosting), but it will also use cavities of canopy trees, foliage, tree bark, crevices on cliffs, and other structures (Fenton and Barclay 1980b, Slough 2009, Coleman and Barclay 2011, Randall et al. 2014).
Maternity colonies may include hundreds of females with young. Females show a strong tendency to roost in large-diameter trees, although roost properties may vary significantly throughout the summer (Olson & Barclay 2013). Females are thought to select a preferred maternity roost at the expense of travelling longer distances to forage, possibly indicative of a limited number of suitable maternity roosting sites (Broders et al. 2006, Randall et al. 2014). Female Little Brown Myotis show a relatively high degree of philopatry
Males roost individually or in small groups and periodically switch roosts. In Quebec, males switched roosts approximately every 2 days (Fabianek et al. 2015). Males use a variety of roost structures, including buildings, rock crevices, foliage, raised bark, and tree cavities (Huynh 2009, Randall et al. 2014). In New Brunswick and Quebec, male Little Brown Myotis primarily roosted in coniferous or conifer-dominated mixedwood stands with a large number of snags (Broders and Forbes 2004, Fabianek et al. 2015).
Northern Myotis
Northern Myotis roost singly or in small groups and favour tree roosts (under raised bark and in tree cavities and crevices), but they can also be found in anthropogenic structures (e.g., under shingles) (Sasse and Perkins 1996, Foster and Kurta 1999, Caceres and Barclay 2000, Carter and Feldhamer 2005).
Northern Myotis’ maternity roosts are strongly associated with forest cover, streams, and tree characteristics (e.g., species, height, diameter, age, and decay) (Caceres and Barclay 2000, Broders and Forbes 2004, Broders et al. 2006). Females prefer to roost in tall, large diameter trees in early- to mid-stages of decay (Sasse and Perkins 1996, Caceres and Barclay 2000, Silvis et al. 2015a). Maternity colonies in Newfoundland and Labrador, Nova Scotia, and New Brunswick were generally in larger-than-average trees (Broders and Forbes 2004, Garroway and Broders 2008, Park and Broders 2012). In New Brunswick and Prince Edward Island, female Northern Myotis primarily roosted in trees in the mid-stages of decay within mature, shade-tolerant deciduous stands (Broders and Forbes 2004, Henderson and Broders 2008). Broders and Forbes (2004) attributed this preference to these tree species’ susceptibility to limb breakage and decay (creating available habitat for roosting), long-lived characteristics (permitting repeated use by bats), and their upland habitats with increased solar radiation (reducing energy costs to maintain the bat’s body temperature). Female Northern Myotis are more likely to resort to anthropogenic structures where habitat is fragmented and few potential roost trees exist (Henderson and Broders 2008). In Nova Scotia, Northern Myotis maternity colonies consisted of females with a high degree of maternal relatedness, likely caused by female philopatry (Patriquin et al. 2013). Females switch maternity roost trees approximately every 1-5 days, but roosts are commonly clustered in roosting areas (Sasse and Perkins 1996, Caceres and Barclay 2000, Carter and Feldhamer 2005, Broders et al. 2006, Olson 2011). The largest roosting area recorded in Canada was 300 ha in Alberta (Olson 2011).
Males generally roost alone under raised bark or within cavities of trees in mid-stages of decay (Broders and Forbes 2004). In New Brunswick and Quebec, male Northern Myotis roosted in coniferous or conifer-dominated mixedwood stands within the study area (Broders and Forbes 2004, Fabianek et al. 2015). In Quebec, males switched roosts approximately every 2 days (Fabianek et al. 2015).
Tri-colored Bat
Less is known about roosts of Tri-colored Bats. Most roost sites are found within forested habitats, where this species also forages. Tri-colored Bats may roost in clumps of dead foliage and lichens (Veilleux et al. 2003, Perry and Thill 2007, Poissant et al. 2010). In Nova Scotia, 30 radio-tagged bats had roosts in large clumps of arboreal lichens (Usnea spp.) that grew on coniferous or deciduous trees relatively close to water features (Poissant et al. 2010).
Females roost alone or in small colonies. In Nova Scotia, as many as 18 Tri-colored Bats were found in a cluster (Poissant et al. 2010). In more anthropogenically-modified landscapes, maternity roosts may be barns or similar human-made structures (Fujita and Kunz 1984). In Nova Scotia, Tri-colored Bats exhibit fidelity to small (<78 ha) roosting areas within and between years (Poissant 2009). In Indiana, females returned to the same area (0.4 ha) each summer and used the same 4-6 trees each year, suggesting value in familiar (and possibly limited) structures (Veilleux and Veilleux 2004).
Males roost individually (Veilleux and Veilleux 2004, Perry and Thill 2007, Poissant 2009) . A single male tracked in Nova Scotia roosted alone in arboreal lichen (Usnea trichodea) (Poissant 2009). In Arkansas, males preferentially roosted in dead leaves of oak trees (Quercus spp.) in sites with less canopy cover, more midstory hardwoods, and more overstory large pines than randomly available (Perry and Thill 2007).
Foraging needs
Little Brown Myotis, Northern Myotis, and Tri-colored Bat are insect predators and will exploit locally abundant patches of prey that may be temporally and spatially scattered. Identification of foraging areas for bats is complicated by sex biases, differences between species, seasonal variations of habitat use by females (e.g., pregnant, lactating, or non-reproducing), and foraging habitat availability and configuration (Henry et al. 2002, Owen et al. 2003, Broders et al. 2006, Randall et al. 2014).
Little Brown Myotis
Little Brown Myotis feed nocturnally on insects (e.g., moths, mayflies, flies, beetles, and caddisflies) and spiders (Moosman et al. 2012, Thomas et al. 2012, Clare et al. 2014). Nevertheless, the diet of Little Brown Myotis can vary significantly based on seasonal, geographic, and environmental factors (Moosman et al. 2012, Clare et al. 2014). On a successful night during peak summer activity, males eat approximately half of their body weight and lactating females may eat their entire body weight in insects (Anthony and Kunz 1977). Peak foraging activity occurs several hours after dusk and often again before sunrise (Fenton 1970, Kunz 1973, Broders et al. 2003). In northern areas (above 60°N) where summer night length is short, pregnant females appeared to alter their foraging behavior by exhibiting only one bout of peak activity. In addition, they foraged for fewer hours than their southerly counterparts though they compensated for reduced time foraging by exhibiting a higher rate of insect capture (Talerico 2008, Reimer 2013).
Foraging Little Brown Myotis are most often associated with open habitats, such as ponds and roads and open canopy (0-50%) forests (Segers and Broders 2014), but have also been recorded gleaning
Northern Myotis
Northern Myotis feed on insects (e.g., moths, beetles, wasps, and flies) and spiders (Lacki et al. 2009, Dodd et al. 2012, Thomas et al. 2012) that are primarily terrestrial in origin (Broders et al. 2014). Unlike Little Brown Myotis, which most often forage over water and are aerial hawkers, Northern Myotis forage more frequently along and within forests and although they feed on flying insects, they also glean prey (Caceres and Barclay 2000, Ratcliffe and Dawson 2003).
Female Northern Myotis foraged along forest-covered creeks in Prince Edward Island (Henderson and Broders 2008). In West Virginia, female Northern Myotis mainly foraged in 70-90 year-old hardwood stands with road corridors (Owen et al. 2003), and in Kentucky, Northern Myotis were found foraging along ridges and midslopes, rather than lower slopes (Lacki et al. 2009). In an intensively managed forest of West Virginia, the mean home range for lactating or pregnant Northern Myotis was 65 ha (Owen et al. 2003). In New Brunswick, Broders et al. (2006) found males and females travel significantly different distances between roost sites and foraging areas. The distance travelled by females between successive roosts was twice as far as males on average (457 m vs. 158 m) (Broders et al. 2006). The authors suggested that females travelled farther because suitable maternity sites were located in poor foraging habitat (Broders et al. 2006).
Tri-colored Bat
Similar to Little Brown Myotis, Tri-colored Bats feed on insects (e.g., flies, beetles, wasps, and moths) after dusk and before dawn using echolocation (Fujita and Kunz 1984, Naughton 2012). Each night, males consume at least half of their body weight in insects and pregnant and nursing females may eat more than their body weight (Naughton 2012).
Foraging predominately occurs in forested riparian areas, over water (e.g., ponds and rivers), and in relatively open areas (Ethier and Fahrig 2011). In Nova Scotia, Farrow and Broders (2011) found Tri-colored Bats foraging at river sites, but found more activity in areas with greater forest cover at a landscape scale, suggesting that this species may avoid landscapes that are cleared for agriculture, urban development, and forest harvesting. The distances between roost sites and foraging areas are generally unknown, but in some locations may be up to 5 km (Quinn and Broders 2007).
Migration
Little Brown Myotis, Northern Myotis, and Tri-colored Bat are considered short-distance migrants, radiating annually from overwintering areas to summering areas in any direction (Fraser et al. 2012, COSEWIC 2013). In Manitoba and Ontario, Little Brown Myotis migrated regionally 35 to 554 km (median 463 km) (Fenton 1970, Dubois and Monson 2007, Norquay et al. 2013). Migratory movements by Northern Myotis are not well understood, but are likely similar to the Little Brown Myotis. Tri-colored Bat have been recorded moving 53-780 km (Griffin 1940, COSEWIC 2013). In addition, Fraser et al. (2012) found that some Tri-colored Bats engage in annual latitudinal migrations, especially those at the northern extent of the range, which may be related to their need to keep warm since they often hibernate individually (COSEWIC 2013). This is further supported by Thorne (2015), who found increased detections of Tri-colored Bats later in the season (i.e., August – September) on islands in the Great Lakes of Ontario.
As noted above, swarming sites may serve as migratory stopover locations (Fenton 1969) and are likely used annually (Rydell et al. 2014). When travelling over large waterbodies, peninsulas and islands may function as stopover sites (Dzal et al. 2009, Thorne 2015). For example, Tri-colored Bats may use Amherst Island, Lake Ontario and Long Point, Lake Erie for migration and stopover (Dzal et al. 2009, Thorne 2015).
Limiting factors
All three species are long-lived and females produce only one (Little Brown Myotis and Northern Myotis) or two (Tri-colored Bat) young annually. Species that are long-lived with low reproductive rates rely on high adult survival rates to maintain populations and thus increases in adult mortality rates heighten the vulnerability of these bat populations. In addition, the first year survival is low (0.23 to 0.46 for Little Brown Myotis) (Frick et al. 2010b). In a recent pre-WNS study from New Hampshire, the annual population growth rate of Little Brown Myotis over 16 years was estimated to be 1.008 (Frick et al. 2010b). In 22 subpopulations in the northeastern U.S, the population growth rate was estimated to be 0.98-1.2 (Frick et al. 2010a). Similarly, the population growth rates of Northern Myotis and Tri-colored Bat were estimated to be 1.03 and 1.04, respectively (Langwig et al. 2012). Predicted population growth rates for Little Brown Myotis in the northeastern U.S. post-WNS was 0.95 (Maslo et al. 2015).
These species are socially gregarious which increases their susceptibility to the spread of diseases (such as WNS) (Langwig et al. 2012). In some areas (e.g., Eastern Canada), these species congregate in large numbers within hibernacula, making them particularly vulnerable to disturbance events (physical or otherwise), It is unknown whether the colonial and social nature of these species creates subpopulation or colony size thresholds below which the survival or reproductive success of individuals decline and/or the population will be unable to recover.
It is also unknown if available overwintering sites with suitable microclimatic conditions are limiting in Canada.
4 Threats
4.1 Threat assessment
The Little Brown Myotis, Northern Myotis, Tri-colored Bat threat assessments are based on the IUCN-CMP (World Conservation Union–Conservation Measures Partnership) unified threats classification system. Threats are defined as the proximate activities or processes that have caused, are causing, or may cause in the future the destruction, degradation, and/or impairment of the entity being assessed (population, species, community, or ecosystem) in the area of interest (global, national, or subnational). For these species, the threat impacts were calculated (%) for the next 10 to 15 years. These numbers further define the IUCN-CMP classification of threat impacts, which are a qualitative median rate of population reduction or area decline for each combination of scope and severity.
It is recognized that numerous knowledge gaps related to bat population-level response to specific threats exist and there is uncertainty associated with severity and scope for some of the identified threats. In areas where bat populations have significantly declined as a result of WNS, it is important to note that the additive mortality
Limiting factors are not considered during this assessment process. For purposes of threat assessment, only present and future threats are considered. Historical threats, indirect or cumulative effects of the threats, or any other relevant information that would help understand the nature of the threats are presented in the Description of Threats section.
Threats for the three species of bats were assessed separately and at a national scale (Tables 2-4). Due to the large geographic range of the three bat species in Canada, the ecological differences in the overwintering behaviour of the Myotis species of bats in the west and the non-random spatial distribution of the threat themselves, it invariably follows that the impacts on bat species and local populations vary across the country. Based on these factors, it may be of value for regions and/or jurisdictions to conduct a threat calculator at a more local scale to obtain a finer resolution on the threats for management purposes.
Threat #a | Threat description | Impactb | Scopec | Severityd | Timinge |
---|---|---|---|---|---|
1 | Residential & commercial development | 0.1-3% Low |
Small (1-10%) |
Moderate (11-30%) |
High (Continuing) |
1.1 | Housing & urban areas | 0.1-3% Low |
Small (1-10%) |
Moderate (11-30%) |
High (Continuing) |
1.2 | Commercial & industrial areas | 0.1-3% Low |
Small (1-10%) |
Moderate (11-30%) |
High (Continuing) |
1.3 | Tourism & recreation areas | <1% Negligible |
Negligible (<1%) |
Serious - Moderate (11-70%) |
High (Continuing) |
2 | Agriculture & aquaculture | 0.1-3% Low |
Restricted (11-30%) |
Slight (1-10%) |
High (Continuing) |
2.1 | Annual & perennial non-timber crops | 0.1-3% Low |
Restricted (11-30%) |
Slight (1-10%) |
High (Continuing) |
3 | Energy production & mining | 8-70% High |
Pervasive (71-100%) |
Serious - Moderate (11-70%) |
High (Continuing) |
3.1 | Oil & gas drilling | 0.1-1% Low |
Small (1-10%) |
Slight - Negligible (0-10%) |
High (Continuing) |
3.2 | Mining & quarrying | 0.1-9% Low |
Restricted - Small (1-30%) |
Moderate (11-30%) |
High (Continuing) |
3.3 | Renewable Energy | 8-70% High |
Pervasive (71-100%) |
Serious - Moderate (11-70%) |
High (Continuing) |
4 | Transportation & service corridors | 0.7-10% Low |
Pervasive (71-100%) |
Slight (1-10%) |
High (Continuing) |
4.1 | Roads & railroads | 0.7-10% Low |
Pervasive (71-100%) |
Slight (1-10%) |
High (Continuing) |
4.2 | Utility & service lines | 0.1-1% Low |
Small (1-10%) |
Slight - Negligible (0-10%) |
High (Continuing) |
5 | Biological resource use | 0.1-7% Low |
Small (1-10%) |
Serious - Moderate (11-70%) |
High (Continuing) |
5.1 | Hunting & collecting terrestrial animals | 0.1-3% Low |
Small (1-10%) |
Moderate (11-30%) |
High (Continuing) |
5.3 | Logging & wood harvesting | 0.1-3% Low |
Small (1-10%) |
Moderate - Slight (1-30%) |
High (Continuing) |
5.4 | Fishing & harvesting aquatic resources | <1% Negligible |
Small (1-10%) |
Negligible (<1%) |
High (Continuing) |
6 | Human intrusions & disturbance | 0.1-3% Low |
Small (1-10%) |
Moderate - Slight (1-30%) |
High (Continuing) |
6.1 | Recreational activities | 0.1-3% Low |
Small (1-10%) |
Moderate - Slight (1-30%) |
High (Continuing) |
6.3 | Work & other activities | <1% Negligible |
Negligible (<1%) |
Slight - Negligible (0-10%) |
High (Continuing) |
7 | Natural system modifications | 0.1-3% Low |
Small (1-10%) |
Moderate - Slight (1-30%) |
High (Continuing) |
7.1 | Fire & fire suppression | 0.1-3% Low |
Small (1-10%) |
Moderate - Slight (1-30%) |
High (Continuing) |
7.2 | Dams & water management/use | <1% Negligible |
Negligible (<1%) |
Extreme - Serious (31-100%) |
High (Continuing) |
8 | Invasive & other problematic species & genes | 22-100% Very High |
Pervasive - Large (31-100%) |
Extreme (71-100%) |
High (Continuing) |
8.1 | Invasive non-native/alien species | 22-100% Very High |
Pervasive - Large (31-100%) |
Extreme (71-100%) |
High (Continuing) |
8.2 | Problematic native species | <1% Negligible |
Negligible (<1%) |
Slight - Negligible (0-10%) |
High (Continuing) |
9 | Pollution | 0.3-21% Medium |
Large (31-70%) |
Moderate - Slight (1-30%) |
High (Continuing) |
9.2 | Industrial & military effluents | 0-1% Low |
Small (1-10%) |
Slight - Negligible (0-10%) |
High (Continuing) |
9.3 | Agricultural & forestry effluents | 0.3-21% Medium |
Large (31-70%) |
Moderate - Slight (1-30%) |
High (Continuing) |
9.5 | Air-borne pollutants | 0-10% Low |
Pervasive (71-100%) |
Slight - Negligible (0-10%) |
High (Continuing) |
9.6 | Excess energy | 0-7% Low |
Large (31-70%) |
Slight - Negligible (0-10%) |
High (Continuing) |
11 | Climate change & severe weather | Unknown | Unknown | Unknown | High (Continuing) |
11.1 | Habitat shifting & alternation | Unknown | Unknown | Unknown | High (Continuing) |
11.2 | Droughts | Unknown | Unknown | Unknown | High (Continuing) |
11.3 | Temperature extremes | Unknown | Unknown | Unknown | High (Continuing) |
11.4 | Storms & flooding | Unknown | Unknown | Unknown | High (Continuing) |
a Threat numbers are provided for Level 1 threats (i.e., whole numbers) and Level 2 threats (i.e., numbers with decimals).
b Impact – The degree to which a species is observed, inferred, or suspected to be directly or indirectly threatened in the area of interest. The impact of each threat is based on severity and scope rating and considers only present and future threats. Threat impact reflects a reduction of a species population or decline/degradation of the area of an ecosystem. The calculated percentage rate of reduction of a species population is provided in addition to the qualitative median rate of population reduction or area decline. The median rate population reduction or area decline for each combination of scope and severity corresponds to the following classes of impact: Very High (75% declines), High (40 %), Medium (15%), and Low (3%). Unknown is used when impact cannot be determined (e.g., if values for either scope or severity are unknown); Not Calculated is used when threat is outside the assessment time (e.g., timing is insignificant/negligible [past threat] or low [possible threat in long term]); Negligible is used when scope or severity is negligible; Not a Threat is used when severity is scored as neutral or potential benefit.
c Scope – Proportion of the species that can reasonably be expected to be affected by the threat within 10 years. Usually measured as a proportion of the species’ population in the area of interest. (Pervasive = 71–100%; Large = 31–70%; Restricted = 11–30%; Small = 1–10%; Negligible < 1%).
d Severity – Within the scope, the level of damage to the species from the threat that can reasonably be expected to be affected by the threat within a 10-year or 3-generation timeframe. Usually measured as the degree of reduction of the species’ population. (Extreme = 71–100%; Serious = 31–70%; Moderate = 11–30%; Slight = 1–10%; Negligible < 1%; Neutral or Potential Benefit ≥ 0%).
e Timing – High = continuing; Moderate = only in the future (could happen in the short term [< 10 years or 3 generations]) or now suspended (could come back in the short term); Low = only in the future (could happen in the long term) or now suspended (could come back in the long term); Insignificant/Negligible = only in the past and unlikely to return, or no direct effect but limiting.
Threat #f | Threat description | Impactg | Scopeh | Severityi | Timingj |
---|---|---|---|---|---|
1 | Residential & commercial development | <1% Negligible |
Negligible (<1%) |
Serious - Moderate (11-70%) |
High (Continuing) |
1.1 | Housing & urban areas | <1% Negligible |
Negligible (<1%) |
Moderate (11-30%) |
High (Continuing) |
1.2 | Commercial & industrial areas | <1% Negligible |
Negligible (<1%) |
Moderate (11-30%) |
High (Continuing) |
1.3 | Tourism & recreation areas | <1% Negligible |
Negligible (<1%) |
Serious - Moderate (11-70%) |
High (Continuing) |
2 | Agriculture & aquaculture | 0.1-3% Low |
Restricted (11-30%) |
Slight (1-10%) |
High (Continuing) |
2.1 | Annual & perennial non-timber crops | 0.1-3% Low |
Restricted (11-30%) |
Slight (1-10%) |
High (Continuing) |
3 | Energy production & mining | 1-30% Medium |
Pervasive (71-100%) |
Moderate - Slight (1-30%) |
High (Continuing) |
3.1 | Oil & gas drilling | 0-1% Low |
Small (1-10%) |
Slight - Negligible (0-10%) |
High (Continuing) |
3.2 | Mining & quarrying | 0.1-9% Low |
Restricted - Small (1-30%) |
Moderate (11-30%) |
High (Continuing) |
3.3 | Renewable Energy | 1-30% Medium |
Pervasive (71-100%) |
Moderate - Slight (1-30%) |
High (Continuing) |
4 | Transportation & service corridors | 0.3-7% Low |
Large (31-70%) |
Slight (1-10%) |
High (Continuing) |
4.1 | Roads & railroads | 0.3-7% Low |
Large (31-70%) |
Slight (1-10%) |
High (Continuing) |
4.2 | Utility & service lines | 0-1% Low |
Small (1-10%) |
Slight - Negligible (0-10%) |
High (Continuing) |
5 | Biological resource use | 0.1-9% Low |
Restricted (11-30%) |
Moderate - Slight (1-30%) |
High (Continuing) |
5.1 | Hunting & collecting terrestrial animals | <1% Negligible |
Negligible (<1%) |
Moderate (11-30%) |
High (Continuing) |
5.3 | Logging & wood harvesting | 0.1-9% Low |
Restricted (11-30%) |
Moderate - Slight (1-30%) |
High (Continuing) |
5.4 | Fishing & harvesting aquatic resources | <1% Negligible |
Small (1-10%) |
Negligible (<1%) |
High (Continuing) |
6 | Human intrusions & disturbance | 0.1-3% Low |
Small (1-10%) |
Moderate - Slight (1-30%) |
High (Continuing) |
6.1 | Recreational activities | 0.1-3% Low |
Small (1-10%) |
Moderate - Slight (1-30%) |
High (Continuing) |
6.3 | Work & other activities | <1% Negligible |
Negligible (<1%) |
Slight - Negligible (0-10%) |
High (Continuing) |
7 | Natural system modifications | 0.1-3% Low |
Small (1-10%) |
Extreme - Serious (31-100%) |
High (Continuing) |
7.1 | Fire & fire suppression | 0.1-3% Low |
Small (1-10%) |
Moderate - Slight (1-30%) |
High (Continuing) |
7.2 | Dams & water management/use | <1% Negligible |
Negligible (<1%) |
Extreme - Serious (31-100%) |
High (Continuing) |
8 | Invasive & other problematic species & genes | 22-100% Very High |
Pervasive - Large (31-100%) |
Extreme (71-100%) |
High (Continuing) |
8.1 | Invasive non-native/alien species | 22-100% Very High |
Pervasive - Large (31-100%) |
Extreme (71-100%) |
High (Continuing) |
8.2 | Problematic native species | <1% Negligible |
Negligible (<1%) |
Slight - Negligible (0-10%) |
High (Continuing) |
9 | Pollution | 0.3-21% Medium |
Pervasive (71-100%) |
Moderate - Slight (1-30%) |
High (Continuing) |
9.2 | Industrial & military effluents | 0-1% Low |
Small (1-10%) |
Slight - Negligible (0-10%) |
High (Continuing) |
9.3 | Agricultural & forestry effluents | 0.3-21% Medium |
Large (31-70%) |
Moderate - Slight (1-30%) |
High (Continuing) |
9.5 | Air-borne pollutants | 0-10% Low |
Pervasive (71-100%) |
Slight - Negligible (0-10%) |
High (Continuing) |
9.6 | Excess energy | 0-7% Low |
Large (31-70%) |
Slight - Negligible (0-10%) |
High (Continuing) |
11 | Climate change & severe weather | Unknown | Unknown | Unknown | High (Continuing) |
11.1 | Habitat shifting & alternation | Unknown | Unknown | Unknown | High (Continuing) |
11.2 | Droughts | Unknown | Unknown | Unknown | High (Continuing) |
11.3 | Temperature extremes | Unknown | Unknown | Unknown | High (Continuing) |
11.4 | Storms & flooding | Unknown | Unknown | Unknown | High (Continuing) |
f Threat numbers are provided for Level 1 threats (i.e., whole numbers) and Level 2 threats (i.e., numbers with decimals).
g Impact – The degree to which a species is observed, inferred, or suspected to be directly or indirectly threatened in the area of interest. The impact of each threat is based on severity and scope rating and considers only present and future threats. Threat impact reflects a reduction of a species population or decline/degradation of the area of an ecosystem. The calculated percentage rate of reduction of a species population is provided in addition to the qualitative median rate of population reduction or area decline. The median rate of population reduction or area decline for each combination of scope and severity corresponds to the following classes of impact: Very High (75% declines), High (40 %), Medium (15%), and Low (3%). Unknown is used when impact cannot be determined (e.g., if values for either scope or severity are unknown); Not Calculated is used when threat is outside the assessment time (e.g., timing is insignificant/negligible [past threat] or low [possible threat in long term]); Negligible is used when scope or severity is negligible; Not a Threat is used when severity is scored as neutral or potential benefit.
h Scope – Proportion of the species that can reasonably be expected to be affected by the threat within 10 years. Usually measured as a proportion of the species’ population in the area of interest. (Pervasive = 71–100%; Large = 31–70%; Restricted = 11–30%; Small = 1–10%; Negligible < 1%).
i Severity – Within the scope, the level of damage to the species from the threat that can reasonably be expected to be affected by the threat within a 10-year or 3-generation timeframe. Usually measured as the degree of reduction of the species’ population. (Extreme = 71–100%; Serious = 31–70%; Moderate = 11–30%; Slight = 1–10%; Negligible < 1%; Neutral or Potential Benefit ≥ 0%).
j Timing – High = continuing; Moderate = only in the future (could happen in the short term [< 10 years or 3 generations]) or now suspended (could come back in the short term); Low = only in the future (could happen in the long term) or now suspended (could come back in the long term); Insignificant/Negligible = only in the past and unlikely to return, or no direct effect but limiting.
Threat #k | Threat description | Impactl | Scopem | Severityn | Timingo |
---|---|---|---|---|---|
1 | Residential & commercial development | 0.1-3% Low |
Small (1-10%) |
Serious - Moderate (11-70%) |
High (Continuing) |
1.1 | Housing & urban areas | 0.1-3% Low |
Small (1-10%) |
Moderate (11-30%) |
High (Continuing) |
1.2 | Commercial & industrial areas | <1% Negligible |
Negligible (<1%) |
Moderate (11-30%) |
High (Continuing) |
1.3 | Tourism & recreation areas | <1% Negligible |
Negligible (<1%) |
Serious - Moderate (11-70%) |
High (Continuing) |
2 | Agriculture & aquaculture | 0.1-3% Low |
Restricted (11-30%) |
Slight (1-10%) |
High (Continuing) |
2.1 | Annual & perennial non-timber crops | 0.1-3% Low |
Restricted (11-30%) |
Slight (1-10%) |
High (Continuing) |
3 | Energy production & mining | 1-30% Medium |
Pervasive (71-100%) |
Moderate (11-30%) |
High (Continuing) |
3.1 | Oil & gas drilling | 0-1% Low |
Small (1-10%) |
Slight - Negligible (0-10%) |
High (Continuing) |
3.2 | Mining & quarrying | 0.1-9% Low |
Restricted - Small (1-30%) |
Moderate (11-30%) |
High (Continuing) |
3.3 | Renewable Energy | 1-30% Medium |
Pervasive (71-100%) |
Moderate - Slight (1-30%) |
High (Continuing) |
4 | Transportation & service corridors | 0.7-10% Low |
Pervasive (71-100%) |
Slight (1-10%) |
High (Continuing) |
4.1 | Roads & railroads | 0.7-10% Low |
Pervasive (71-100%) |
Slight (1-10%) |
High (Continuing) |
4.2 | Utility & service lines | 0-1% Low |
Small (1-10%) |
Slight - Negligible (0-10%) |
High (Continuing) |
5 | Biological resource use | 0.1-9% Low |
Small (1-10%) |
Moderate (11-30%) |
High (Continuing) |
5.1 | Hunting & collecting terrestrial animals | <1% Negligible |
Negligible (<1%) |
Moderate (11-30%) |
High (Continuing) |
5.3 | Logging & wood harvesting | 0.1-9% Low |
Restricted (11-30%) |
Moderate - Slight (1-30%) |
High (Continuing) |
5.4 | Fishing & harvesting aquatic resources | <1% Negligible |
Small (1-10%) |
Negligible (<1%) |
High (Continuing) |
6 | Human intrusions & disturbance | 0.1-3% Low |
Small (1-10%) |
Moderate - Slight (1-30%) |
High (Continuing) |
6.1 | Recreational activities | 0.1-3% Low |
Small (1-10%) |
Moderate - Slight (1-30%) |
High (Continuing) |
6.3 | Work & other activities | <1% Negligible |
Negligible (<1%) |
Slight - Negligible (0-10%) |
High (Continuing) |
7 | Natural system modifications | 0.1-3% Low |
Small (1-10%) |
Extreme - Serious (31-100%) |
High (Continuing) |
7.1 | Fire & fire suppression | 0.1-3% Low |
Small (1-10%) |
Moderate - Slight (1-30%) |
High (Continuing) |
7.2 | Dams & water management/use | <1% Negligible |
Negligible (<1%) |
Extreme - Serious (31-100%) |
High (Continuing) |
8 | Invasive & other problematic species & genes | 22-100% Very High |
Pervasive - Large (31-100%) |
Extreme (71-100%) |
High (Continuing) |
8.1 | Invasive non-native/alien species | 22-100% Very High |
Pervasive - Large (31-100%) |
Extreme (71-100%) |
High (Continuing) |
8.2 | Problematic native species | <1% Negligible |
Negligible (<1%) |
Slight - Negligible (0-10%) |
High (Continuing) |
9 | Pollution | 0.3-21% Medium |
Pervasive (71-100%) |
Moderate - Slight (1-30%) |
High (Continuing) |
9.2 | Industrial & military effluents | 0-1% Low |
Small (1-10%) |
Slight - Negligible (0-10%) |
High (Continuing) |
9.3 | Agricultural & forestry effluents | 0.3-21% Medium |
Large (31-70%) |
Moderate - Slight (1-30%) |
High (Continuing) |
9.5 | Air-borne pollutants | 0-10% Low |
Pervasive (71-100%) |
Slight - Negligible (0-10%) |
High (Continuing) |
9.6 | Excess energy | 0-10% Low |
Pervasive (71-100%) |
Slight - Negligible (0-10%) |
High (Continuing) |
11 | Climate change & severe weather | Unknown | Unknown | Unknown | High (Continuing) |
11.1 | Habitat shifting & alternation | Unknown | Unknown | Unknown | High (Continuing) |
11.2 | Droughts | Unknown | Unknown | Unknown | High (Continuing) |
11.3 | Temperature extremes | Unknown | Unknown | Unknown | High (Continuing) |
11.4 | Storms & flooding | Unknown | Unknown | Unknown | High (Continuing) |
k Threat numbers are provided for Level 1 threats (i.e., whole numbers) and Level 2 threats (i.e., numbers with decimals).
l Impact – The degree to which a species is observed, inferred, or suspected to be directly or indirectly threatened in the area of interest. The impact of each threat is based on severity and scope rating and considers only present and future threats. Threat impact reflects a reduction of a species population or decline/degradation of the area of an ecosystem. The calculated percentage rate of reduction of a species population is provided in addition to the qualitative median rate of population reduction or area decline. The median rate of population reduction or area decline for each combination of scope and severity corresponds to the following classes of impact: Very High (75% declines), High (40 %), Medium (15%), and Low (3%). Unknown: used when impact cannot be determined (e.g., if values for either scope or severity are unknown); Not Calculated: impact not calculated as threat is outside the assessment time (e.g., timing is insignificant/negligible [past threat] or low [possible threat in long term]); Negligible: when scope or severity is negligible; Not a Threat: when severity is scored as neutral or potential benefit.
m Scope – Proportion of the species that can reasonably be expected to be affected by the threat within 10 years. Usually measured as a proportion of the species’ population in the area of interest. (Pervasive = 71–100%; Large = 31–70%; Restricted = 11–30%; Small = 1–10%; Negligible < 1%).
n Severity – Within the scope, the level of damage to the species from the threat that can reasonably be expected to be affected by the threat within a 10-year or 3-generation timeframe. Usually measured as the degree of reduction of the species’ population. (Extreme = 71–100%; Serious = 31–70%; Moderate = 11–30%; Slight = 1–10%; Negligible < 1%; Neutral or Potential Benefit ≥ 0%).
o Timing – High = continuing; Moderate = only in the future (could happen in the short term [< 10 years or 3 generations]) or now suspended (could come back in the short term); Low = only in the future (could happen in the long term) or now suspended (could come back in the long term); Insignificant/Negligible = only in the past and unlikely to return, or no direct effect but limiting.
4.2 Description of threats
The overall Canada-wide threat impact for all three species is Very High to High
The primary threat to all three species is Invasive and other problematic species and genes – Invasive non-native/alien species (i.e., WNS). Details are discussed below under the Threat Level 1 headings which are listed here in the order they are presented in Tables 2-4. While scope, severity and impact of each threat were assessed separately for each species, the suite of threats identified is the same and are discussed in combination below.
4.2.1 Residential & commercial development
Housing & urban areas; commercial & industrial areas
Of the three species of bats, Little Brown Myotis most regularly uses buildings and bat boxes for maternity colonies. The number of bat colonies in buildings may be declining as a result of the deterioration (or demolition) of structures and attempts by landowners to exclude bats (Kunz and Reynolds 2003). Newly built structures are often constructed in ways that do not allow bat access or maternity roost establishment.
Renovations or alterations to buildings used as maternity roosts may eliminate access to the roost change the microclimate characteristics (e.g., air flow, temperature) of the roost and/or trap bats inside if alterations are performed when occupied by bats. Because females tend to show a relatively high degree of fidelity, excluding bats from previously occupied maternity roosts in anthropogenic structures would be considered habitat loss, particularly when alternative roosting sites in the area do not exist. Similarly, habitat loss would occur if a previously occupied bat box is removed from a site.
The effects of roost exclusion may depend on the availability of other suitable habitat, timing, bat species, bat sex, and other factors. Roost exclusions may lower reproductive success (especially if exclusion occurs while occupied), alter home range size, change mean colony size, and decrease site fidelity (Brigham and Fenton 1986, Neilson and Fenton 1994, Borkin et al. 2011, Chaverri and Kunz 2011). Little Brown Myotis may abandon roosting areas after being excluded from roost sites (Neilson and Fenton 1994).
Little Brown Myotis maternity roosts are often found in buildings in Canada (Canadian Wildlife Federation 2018). The scope represents only the anticipated proportion of all Little Brown Myotis whose roosts or foraging areas are likely to be impacted by development over the next 10-15 years, which is thought to be small. The severity reflects the anticipated average impact across all affected bats of loss of a roost. In some individual colonies, the impact may be quite severe (e.g., if a maternity roost is destroyed when there are young or adults in the building or there are no alternative roosts available), but, in other cases, the bats may be able to switch to another roost site, with minimal long-term impact, although it is recognized that new housing developments tend not to create suitable roosting habitat for bats to replace roosts lost in upgrades. Overall, across all situations where bats may be impacted, the average impact is unlikely to exceed 30% loss of the population for affected bats over the next 10-15 years and would usually average lower (especially given that situations of deliberate exclusion are not included here, but, rather, under threat 5.1).
Northern Myotis and Tri-colored Bat rarely use anthropogenic structures for establishing maternity roosts and this aspect of the threat is not a concern for these species. However, urban/residential and commercial/industrial development also results in the removal, degradation, and fragmentation of foraging and natural roosting habitat (e.g., forests, wetlands and riparian areas). Impacts of urban expansion and residential development are thought to have a negligible impact on Northern Myotis. In contrast, urban development may be a particularly important consideration for theTri-colored Bat whose more restricted range in Canada overlaps highly-populated areas, including southern Ontario. Ontario’s population is expected to grow by 30%, or almost 4.2 million people, by 2041; most growth is expected in the southern region of the province with the Greater Toronto Area being the fastest growing region of the province (Ontario Ministry of Finance 2016). The impact of commercial and industrial areas on Northern Myotis and Tri-colored Bat species is Negligible, whereas the impact on Little Brown Myotis is likely to result in a 0.1 – 3% population decline.
Tourism & recreation areas
Modifications for tourists (e.g., observation platforms) or the erection of physical barriers (e.g., gates) can cause restricted or altered airflow or modify other microclimatic characteristics of the hibernaculum (U.S. Fish and Wildlife Service 2007). A well-known cave system in Ontario, which was used as a hibernaculum by these three species of bats (Fenton 1969, Thomas et al. 1979), underwent extensive modifications (e.g., lighting, gates/doors at entrances, paved stairways and floor) to turn it into a commercial tourist attraction (Petrick 2015).
Gates are often considered the most efficient and effective technique to control human access to hibernacula, but even so-called bat-friendly gating may cause bats to avoid the hibernaculum, collide with the gate, or cause significant changes in bat behaviour (Spanjer and Fenton 2005, U.S. Fish and Wildlife Service 2007, Derusseau and Huntly 2012, Diamond and Diamond 2014). Gating should be completed using well-supported designs in conjunction with bat monitoring to ensure no negative impacts to bats.
Sealing or blocking the entrance to a hibernaculum can represent a potentially significant source of habitat loss. For example, when a hibernaculum in Kentucky was blocked by a new gift shop, thousands of Indiana Bats (Myotis sodalis) clung to the walls of the building, rather than search for an alternative hibernaculum (Murphy 1987). Even blockages of small entrances can alter airflow patterns and change internal temperatures (U.S. Fish and Wildlife Service 2007).
Only a small proportion (<1%) of the population of each of the three bats species is likely to be affected. Severity can be extreme if development causes disturbance to an active hibernaculum, but given increasing public awareness and education related to bats and the potential for mitigation (e.g., seasonal closures during hibernation season), this would likely be rare. As such, across all future developments, average severity is likely to be much lower, but a broad range has been retained to reflect potential impacts if development happens without mitigation. This category does not include disturbance related to recreational activities in undeveloped caves which is captured under threat 6.1.
4.2.2 Agriculture & aquaculture
Annual & perennial non-timber crops
The removal, degradation, and fragmentation of foraging and natural roosting habitat (e.g., forests, wetlands and riparian areas) can be caused by a variety of anthropogenic sources, including through direct conversion for agricultural use and intensification of agricultural practices. While the rate of agricultural expansion (i.e., conversion of natural habitats to agricultural use) has decreased over the last few decades, a concomitant shift to the intensification of management on land already under agricultural use has occurred (Matson et al. 1997). Intensification of agriculture includes trends such as increased mechanization and use of irrigation, increased field size, planting of high-yield crop varieties (e.g., cereal monocultures) and increased chemical use - all at the expense of woodlots, hedgerows, farm ponds and other marginal habitat from an agronomic point of view (Matson et al. 1997, Bélanger and Grenier 2002, Mineau and Whiteside 2013). These intensively managed agricultural systems result in an overall reduction of biodiversity (Matson et al. 1997).
Historical wetland loss in Canada is estimated at approximately 70% within settled areas, with draining for agriculture accounting for the majority (85%) of known conversions (Haak 2008). Wetlands and areas around waterbodies (e.g., riparian areas and forest edges) are important foraging habitat for Little Brown Myotis, Northern Myotis and Tri-colored Bat. Activities that degrade or remove wetlands have the potential to have negative impacts to foraging habitat availability and quality. Wetland loss in southern Ontario, where all three species occur, has been extensive and continues (additional losses of 3.5% between 1982 and 2002) (Federal Provincial and Territorial Governments of Canada 2010). Within the range of Little Brown Myotis, estimates of wetland losses in the Prairie Pothole region of Canada vary between 40 to 71% (Federal Provincial and Territorial Governments of Canada 2010). Wetland loss and degradation continues within this region mainly due to agricultural intensification.
Land conversion has been intensive in some portions of these species ranges. For example, 73% of the boreal hardwood transition zone in Saskatchewan has been converted to agriculture, with 25% lost between 1966 and 1994 (Hobson et al. 2002). Young et al. (2006) calculated an annual rate of change in forest cover along the southern boreal edge of Alberta to be -0.82% per year. It is estimated that 70% of the woodlands in the St. Lawrence Valley have been removed, primarily in southwestern Quebec and southern Ontario (Freemark and Merriam 1986).
In agriculturally-dominated landscapes, some species follow linear forest features for commuting and foraging (Henderson and Broders 2008). Northern Myotis traveled following a hedgerow of trees in a agriculturally-dominated landscape in Prince Edward Island (Henderson and Broders 2008). Myotis spp. and Tri-colored Bat were not directly observed using open areas (such as cultivated and fallow fields and golf courses) in Quebec, but were active in the wooded areas adjoining these features (Fabianek et al. 2011). Agricultural intensification that removes hedgerows and field margins could be reducing foraging and commuting habitat (Wickramasinghe et al. 2003).
When agricultural activities occur nearby hibernacula they can cause, exacerbate, or accelerate blockages of airflow, create changes to hydrology or microclimatic conditions, potentially cause flooding, or directly degrade the habitat (McAlpine 1983, U.S. Fish and Wildlife Service 2007).
However, in some areas of eastern Canada, reversion of agricultural land to forest may partially offset loss from intensification/ conversion. A significant proportion of the population of each of the three species lives or forages in areas where some agricultural impacts are anticipated, but given current environmental legislation protecting wetlands (which can be particularly important for foraging), that most agricultural impacts only result in partial loss of habitat in an area, and that most populations probably are not currently habitat limited, the average impact across all affected populations is likely to vary between 0.1–3%.
Additional threats associated with agriculture, that are evaluated in other categories, include impacts of agricultural chemicals such as pesticides (listed under 9.3) and risk of spreading diseases such as White Nose Syndrome through use of bat guano as a fertilizer (listed under threat 8.1).
4.2.3 Energy production & mining
Oil & gas drilling
Industrial activities, such as quarrying, mining (exploration and development) and oil and gas (exploration and development) outside of hibernacula can cause, exacerbate, or accelerate blockages of airflow, create changes to hydrology or microclimatic conditions, potentially cause flooding, or directly degrade the habitat (McAlpine 1983, U.S. Fish and Wildlife Service 2007). Mine or cave entrances may collapse if heavy machinery is used near weak areas of the hibernaculum (McAlpine 1983, U.S. Fish and Wildlife Service 2007). Once bats are blocked from entry, the hibernaculum can no longer be used.
Oil and gas exploration and development, through the creation of well pads and narrow linear seismic lines can remove natural habitat cover but can also create edge habitat that could be used for foraging and commuting by the three bat species. This is likely to affect only a small portion of the population of each of the three species, and is likely to have little or no population level impacts.
Mining & quarrying
According to the Mining Association of Canada Facts and Figures report (2016), several new mines are expected to be in operation this year in Canada (Marshall 2016). Rehabilitation activities (e.g., backfilling, removal of head frames) at old mine sites can affect the suitability of hibernacula and disturb the bats using them if activities are carried out during hibernation period. Conversely, the decommissioning of active or semi-active mines (e.g., shutting off water pumps) can result in flooding of hibernacula. Mining companies may reactivate previously abandoned mines for extraction purposes as a result of fluctuations in mineral prices, but there is little information on the frequency of this practice in Canada. The prevalence is assumed to be low, but may be a concern for particular areas of the country.
Many of the known hibernacula in Canada, particularly in the east, are in abandoned mines. While this is the case, it should be noted that mines are one of the first places to look for bats and that most hibernacula across Canada likely remain unknown, particularly in the west. The scope is difficult to predict because activity is tied to commodity prices which are volatile. Given that a proportion of each of the three species use mines for hibernacula, there is a possibility that over 10% of the population could be affected by new mining activity in the next 10–15 years, although it may also be much lower. However, with appropriate provincial/ territorial/ federal legislation/ regulations to protect bats, it is likely that most impacts will be mitigated (e.g., by avoiding activities in areas of mines where bats are hibernating, creation of alternative suitable sites in active mines, and/or sealing mine shafts in a bat friendly manner). As a result, while severe impacts may sometimes happen to an individual colony, average impacts across all affected colonies will be much lower. Oil sands development is also included in this category, but likely to affect <1% of the population and the primary impact will be loss of habitat, which is partially mitigated by restoration. Noise and vibration are captured in threat 9.6, while mining effluent is covered under threat 9.2.
Renewable energy
Bats can be killed either through direct collisions with turbine blades (Horn et al. 2008) or barotrauma caused by the sudden drop in air pressure behind the blades (Baerwald et al. 2008, Grodsky et al. 2011, Rollins et al. 2012). Wind turbines represent one of the largest sources of anthropogenic mortality documented for bats (Cryan and Brown 2007, Cryan 2011, O'Shea et al. 2016; Zimmerling and Francis 2016). Results from mortality studies at various sites in the United States and Europe suggest that annual bat mortality ranges from 0 to over 50 deaths per turbine, but data collection protocols, experimental design, and analysis methods varied substantially among wind farms (Kunz et al. 2007, Arnett et al. 2008, Cryan 2011, Hayes 2013, Smallwood 2013).
Within Canada, impacts of wind energy development can vary substantially across the ranges of the species. At one wind power facility in Ontario, bat mortality was dominated by Little Brown Myotis; this species accounted for 46% of all bat mortality in July and 38% over the period of April to September (OMNRF, unpublished data). At a wind power facility in British Columbia, Little Brown Myotis and Northern Myotis comprised 44% of all bat mortalities recorded with most fatalities occurring in July and August (Hemmera 2011).
Using data pre- and post-WNS from 64 wind farms across Canada, it is estimated that 15.5 ± 3.8 bats per turbine are killed annually (Zimmerling and Francis 2016). Based on 4019 installed turbines (the number installed in Canada as of December 2013), an estimated 47,400 bats are killed per year (95% C.I.
It is unknown if Northern Myotis and Tri-colored Bat are less vulnerable to impacts from wind turbines because of differences in flight, foraging behavior, or habitat, or if they simply have smaller populations and are therefore generally uncommon around wind farms. Nevertheless, even low rates of mortality have the potential to be biologically significant for relatively rare species; it is possible that future wind farms, if inadvertently located near important concentration areas such as along migration routes or near maternity roosts, swarming sites or hibernacula, could cause high mortality. In WNS affected areas, the biological significance of any mortality has the potential to impact the ability of local populations to recover and/or develop resistance to the fungus. Mortality rates are anticipated to increase as the number of turbines increase.
Presently, mitigation measures to reduce overall bat mortality related to wind turbine development may include the feathering of wind turbine blades or increasing the cut-in speed when the risk to bats is particularly high (e.g., at night during peak migration) (Baerwald et al. 2009). Baerwald et al. (2009) demonstrated that these mitigation techniques reduced bat fatalities by approximately 60% at a site in southwestern Alberta. Arnett et al. (2009, 2013b) found that increasing the speed at which the turbine starts to rotate and generate power reduced bat mortalities by approximately 73% (range 44% to 93%) at a wind farm in Pennsylvania with a marginal (~1%) loss in annual power. However, recent research suggests that increasing the cut-in speed to 5.5 m/sec may not be effective at mitigating Myotis mortality (Zimmerling et al. 2016). Smart curtailment is a general term referring to any curtailment strategy that relies on more variables than the local wind speeds to determine optimal periods of curtailment to minimize potential impacts to a target species, or species group. A recent study (Sutter et al. 2016) on the U.S. mainland demonstrated that some smart curtailment systems are effective in reducing fatalities of Little Brown Myotis by as much as 90% when combined with feathering below the cut-in speed. A combination of real-time exposure (bat activity at the hub) as determined through the use of ultrasonic detectors and real-time weather data from the meteorological tower was used to identify when there was a high risk of fatalities, and turbines were curtailed so that they had a rotation of less than 2 rpms only when this risk was high. The use of ultrasonic broadcasts may also reduce bat fatalities at wind turbines by deterring bats from approaching the sound source (Arnett et al. 2013a), but their effectiveness at reducing Myotis mortality has not been rigorously assessed. In some circumstances, operational mitigation techniques may include the periodic shutdown of select turbines during the highest risk periods.
Scope is based on proportion of bats that are within 450 km of existing or anticipated wind turbines over the next 10 years, based on the average distance (450 km) that Little Brown Myotis migrate from maternity sites to hibernacula based on telemetry studies. Similar values have been reported for Tri-colored Bat. Although there are few data for Northern Myotis, there seasonal movements are likely similar to Little Brown Myois. Severity considers that, based on Zimmerling & Francis (2016), up to 1.4%/year of the Little Brown Myotis population may be killed, which could increase to 5%/year over the next 15-30 years with projected growth in number of turbines (much less severity is predicted for Northern Myotis and Tri-colored Bat). However, there is considerable uncertainty in that estimate, due to uncertainty in the starting population sizes. There is also uncertainty as to the extent to which this mortality would be additive (reducing the population) versus compensatory (offsetting other sources of mortality). Given that adult Little Brown Myotis normally have relatively high survival rates (80-90%), a substantial portion of this mortality could be additive. If totally additive, this could have an impact up to a 44% reduction in populations over 15 years (and higher over 30 years). However, some is likely compensatory, so the actual impact may be lower, hence the uncertainty range. The solar component of this threat is likely to be a negligible scope over next 10 years, with low severity, but it is acknowledged that this is an emerging threat that is difficult to predict.
4.2.4 Transportation & service corridors
Road & railroads
Roads can pose a risk to bat populations through direct mortality from collisions with vehicles as well as through changes to habitat. Seasonal timing, surrounding habitat, and level of vehicular traffic affect the number of collisions with vehicles (Lesiński et al. 2011, Medinas et al. 2013). Many bat species forage in edge habitat such as those provided along roads (Grindal 1996) and Little Brown Myotis and Northern Myotis will often use roads for commuting (Limpens et al. 1989). Mortality rates are highest near roosts and active foraging areas (Medinas et al. 2013) and low-flying species, such as Northern Myotis and Tri-colored Bat, appear to be more vulnerable (Abbott et al. 2015, Fensome and Mathews 2016). A review of the information available for European bat species showed juvenile bats at a higher risk of direct mortality from vehicles than adults, and that males were more at risk than females (Fensome and Mathews 2016). The authors suggest young bats may be more at risk because they are less experienced and maneuverable flyers than adults, and that males tend to disperse greater distances than females, putting them in contact with roads more often (Fensome and Mathews 2016).
Russell et al. (2009) found that Little Brown Myotis crossed roads at the canopy level when forest was available but where canopy cover was low (<6 m), bats crossed the road lower and closer to traffic; when crossing open fields, bats travelled less than 2 m above the ground. They collected 27 road-killed Little Brown Myotis between May and September at their study site which encompassed a travel route from a maternity colony to a nearby foraging area. There are also anecdotal reports of bats colliding with non_traditional/recreational vehicles and devices, such as water crafts (e.g., boats, personal watercrafts, and wind surfers), fishing lines, and all-terrain vehicles. There is no information available on the number of bats killed by collisions with vehicles in Canada and carcasses are often difficult to find or are removed by predators before they can be accounted for.
Roads can also act as barriers to bats by restricting movements between habitats and changing habitat use (Abbott et al. 2012, Bennett and Zurcher 2013, Kitzes and Merenlender 2014, Abbott et al. 2015, Fensome and Mathews 2016). Where canopy cover was lacking nearby the highway, fewer bats were observed crossing the road (Russell et al. 2009). Major roads appear to constrain movement more than secondary roads (Fensome and Mathews 2016) though there is little information available related to how individual species interact with roads.
The high scope reflects the fact that most Little Brown Myotis and Tri-colored Bats, and to a lesser extent Northern Myotis likely encounter roads at some point in their life. The severity represents the fact that there may be significant local impacts, but across all individuals encountering roads, average impacts to the population are probably less than 1%.
Utility & service lines
Utility and service lines, such as hydro corridors and seismic lines, can create forest-edge habitat that affords both foraging and commuting opportunities to bats, in addition to protection from wind and predators. However, there is evidence that bat activity decreases with increasing distance from the tree line into the open area. Verboom and Spoelstra (1999) found Pipistrelle Bat (Pipistrelle pipistrelle) activity decreased further from the tree line (up to 50 m). A study investigating edge use and patches of residual trees use in harvested boreal forests showed Little Brown Myotis and Northern Myotis were least active within the centre of cutblocks, up to a distance of 30 m from any edge (Hogberg et al. 2002). It has been suggested that the use of open areas by vespertilionid bats is constrained by the absence of vertical elements that can be used as landmarks (Verboom and Spoelstra 1999). It may also be related to exposure to predation risk and wind. It is possible that utility and service lines that have a wide swath of open area associated with them may alter bat movement and foraging behaviour, though additional study on this is needed. The scope assumes that relatively few bat populations will be negatively impacted by these activities. The severity reflects the possibility that there may be impacts on affected populations, but also the possibility these impacts could be negligible.
4.2.5 Biological resource use
Hunting & collecting terrestrial animals
Some species of bats, including Little Brown Myotis, often use anthropogenic structures as maternity roosts or hibernacula. Noise, the accumulation of feces (guano), and fears of contracting histoplasmosis
Intentional harm to individuals within hibernacula has also been reported. For example, all bats (~800 Little Brown Myotis and Northern Myotis) were removed for incineration from the only known hibernaculum of Northern Myotis on Prince Edward Island in 1989 (Brown et al. 2007). Although the bats were rescued from incineration, attempts to keep them over winter failed and they all perished (Brown et al. 2007).
Some provinces and territories have taken measures to reduce the risk of intentional harm. For example, New Brunswick Department of Natural Resources has removed these species from the list of nuisance wildlife under the New Brunswick Fish amd Wildlife Act, thereby providing support for non-lethal alternatives in their management. Saskatchewan’s removal of all Chiroptera from the list of unprotected wildlife under The Wildlife Amendment Regulation 2013 protects bats from all harm, providing support for non-lethal alternatives in their management. See section 2: Species Status Information for more legislation protecting individuals.
The impact of this threat on Northern Myotis and Tri-colored Bat is considered Negligible because they rarely establish maternity colonies in human structures.
The impact of this threat is of greater importance to Little Brown Myotis because they routinely establish maternity colonies in human structures. However, only a small proportion of the total population (< 10%) is likely to be evicted over the next 10-15 years. The impacts on individual colonies that are evicted can sometimes be quite severe (e.g., if most adults or young are killed). However, some forms of eviction practices are bat-friendly, and LBM are often able to find alternative roosts if roosts are sealed when bats are absent. With increased education, outreach and regulations protecting bats, the proportion of exclusions resulting in mortality should decrease. Thus, the average impact across all exclusions is unlikely to exceed 30% of affected populations.
Logging & wood harvesting
The removal, degradation, and fragmentation of foraging and roosting habitat (e.g., forests, wetlands and riparian areas) can be caused by a variety of anthropogenic sources, including forestry harvesting. For example, forestry and timber harvesting operations (e.g., salvage logging) may remove tracts of mature forests, as well as individual snags that may be used by male and female bats for roosting.
Activities that result in the removal of trees or forested landscapes have the potential to destroy or degrade natural roosts (maternity and day roosts) for all three bat species. The effects of roost tree removal may depend on the availability of other suitable habitat, timing, bat species, bat sex, and other factors. Roost removal may alter home range size, change mean colony size, and increase travel distances resulting in increased energetic costs (Borkin et al. 2011, Chaverri and Kunz 2011). Depending on habitat availability, bats may use another tree for roosting if a previous maternity roost is removed outside the breeding season (Silvis et al. 2015b). For Northern Myotis maternity roosts in Kentucky, the number of roosts, roost site characteristics, and overall space used did not change after single highly-used roosts and 24% of secondary roosts were experimentally removed prior to the breeding season (Silvis et al. 2015b). However, the distances bats moved between sequential maternity roosts doubled within areas where secondary roosts were removed (Silvis et al. 2015b). Nevertheless, Silvis et al. (2015b) noted that tolerance limits of maternity roost loss may be influenced by local forest conditions and the social / behavioral characteristics of the species using the roost.
The scope reflects the fact that only some of the population of Little Brown Myotis uses forests as roosts (many now roost in houses) and that over the next 10-15 years, no more than 3-7% of forested lands in Canada are likely to be harvested (Natural Resources Canada 2018). In contrast, most of the population of Northern Myotis and Tri-colored Bat use forests as roosts. In terms of severity, within areas that are logged, many bats may be able to find alternative roosts, but some could be killed if logging happens when trees are occupied. In some areas, loss of trees with cavities could limit availability of natural maternity roosts. This may be more likely in areas where forestry is focused on old growth forests that are more likely to have cavities suitable for bats. Clear cuts may also result in some loss of foraging areas, at least until they partially regenerate. Impacts on local populations could potentially be high, but average impacts in affected areas are likely to be lower, especially with appropriate mitigation (e.g., avoiding logging older trees during the breeding season).
Fishing & harvesting aquatic resources
Though not documented in the literature, there are reports of anglers catching bats on fishing hooks. Little Brown Myotis and Tri-colored Bat often forage over water and this behaviour may increase their vulnerability to being caught on fishing lines. Fly-fishing may be of particular concern since the lure mimics a flying and/or dead insect. There are also reports of Little Brown Myotis colliding with the fishing line itself while foraging low over the water. The proportion of Little Brown Myotis, Northern Myotis and Tri-colored Bat foraging in areas and at times where they encounter fishermen is likely to be small, and even if a few bats are killed, this is likely to have a negligible effect on populations.
4.2.6 Human intrusions & disturbance
Recreational activities
Visitation by people (Thomas 1995) or handling of hibernating bats can result in arousal from torpor (Speakman et al. 1991). When in deep torpor, bats are generally unaffected by ambient noise (Harrison 1965), but some individuals may respond to noise and light, arouse from torpor, and begin to fly (Thomas 1995). These individuals can then cause a cascade of arousals in nearby bats, resulting from their tactile activities (e.g., attempted copulation, rejoining the cluster of bats) (Thomas 1995). Even non-intrusive visitations can cause severe fat consumption (premature energy depletion), starvation, reduced energy reserves for reproduction, and death (Gaisler et al. 1981, Boyles and Brack 2009, Olson et al. 2011). The population of Little Brown Myotis significantly increased in an Alberta cave after winter and autumn access was restricted to reduce disturbance during hibernation and swarming (Olson et al. 2011). Disturbance tolerance is related to the length of winter and number and rate of visits; repeated visits over several consecutive days have the most severe impacts (Boyles and Brack 2009). Because bats with WNS have more frequent arousal episodes (Reeder et al. 2012), the additive effect of human-caused arousals within WNS-affected hibernacula may be significant.
Tourists, spelunkers, recreational users, and researchers are the main visitors to hibernacula. Visitation in the summer (when most occur) likely has less direct impact on bats because the site is not being used or bats can replenish fat reserves. To minimize visitation, year-round gates have been installed at some hibernacula, spelunking societies have posted guidelines (e.g., Manitoba Speleological Society) (SSM 2015), and bat researchers have minimized the number and duration of their visits. However, overall most hibernacula are not in areas that are regularly visited by people, many are inaccessible to people, and most visits are likely in the summer, outside the hibernation season. There are some examples of caves where numbers have remained stable and bats have shown tolerance to visitation despite regular disturbance while other examples of declines and/or complete abandonment. Most hibernacula are unlikely to be disturbed by tourists when bats are present (i.e., during the hibernation season) over the next 10-15 years (especially given growing awareness of conservation needs for bats). Among visited caves, impacts may sometimes be severe but, on average, will probably be much lower.
Work & other activities
This threat considers potential impacts of research activities, including collections of individuals, on bat populations. Some past research activities (e.g., banding and research in hibernacula) have been detrimental to bats. Some impacts could be incidental to other types of research happening within areas used by bats (e.g., research on other aspects of caves). With increasing awareness of bat conservation concerns, most bat/cave researchers should be aware of risks of disturbance during hibernation, as well as risks of transmitting White Nose Syndrome, taking appropriate precautions such as decontamination protocols. For research targeting bats, the need to obtain permits and animal care approvals for proposed protocols should reduce the risk of negative impacts. Some research may also lead to improved management of bats, which can have a net benefit, offsetting any impacts to individuals. Overall, in Canada, research is likely to affect a negligible proportion of the national population. Although some studies may have an impact on a local population, on average, across all studies over the next 10-15 years, the impacts of research on populations is likely to be slight to negligible.
4.2.7 Natural system modifications
Fire and fire suppression
Fires differ in their intensity, frequency and extent and in turn shape the resulting availability of habitat (configuration, composition and successional stage) and abundance and distribution of associated wildlife species. As fire increases the heterogeneity of forested landscapes, an increase in the diversity of available habitat for bats is also expected (Fule et al. 2004, Boyles and Aubrey 2006). Early successional forest stages following burns can provide important areas for foraging while older forests continue to be important for roosting (Loeb and O'Keefe 2011). Fire initially results in an increase of dead and dying trees, an increase of light availability and reduction of canopy and sub-canopy tree density – all characteristics which may be beneficial to cavity-roosting bats (Boyles and Aubrey 2006). Forest bat species are presumably well-adapted to dynamic and spatially complex mosaics and presumably have evolved roosting strategies to limit their vulnerability to fire (Carter et al. 2002, Loeb and O'Keefe 2011).
Some studies have shown increases in insect abundance following fire that could increase bat foraging opportunities (Buchalski et al. 2013). Reduction in sub-canopy vegetation can also provide favorable foraging habitat. Fire may also provide roosting habitat in the form of dead and dying trees, though standing snags may also be removed. In forests managed by prescribed burns in Missouri, the number of roosting sites for Evening Bats (Nycticeius humeralis), which roost in tree cavities, increased (Boyles and Aubrey 2006). A study in Kentucky showed Northern Myotis to be tolerant of prescribed fire; the bats responded to fire by shifting foraging locations and roost selection (Lacki et al. 2009).
Spring burns have the potential to disturb bats when maternity colonies are being established, particularly when non-volant young are present. However, bats may be capable of escaping tree roosts before exposure to heat and gases/smoke occurs (Rodrigue et al. 2001, Dickenson et al. 2009), although this may not always be possible. High temperatures along the leading of a fire can pose a risk to bats through direct burning and ear damage (Dickenson et al. 2009). Fires that are particularly intense and fast-moving, such as predicted with climate change, pose a greater risk.
On average, the actual area of forest burned each year in Canada exceeds the area logged, but not necessarily as often in areas with high densities of bats (Natural Resource Canada 2018). Thus, over a 10-15 year period, no more than 1-10% of the population may be affected by burns. While severe fires may sometimes cause a moderate impact to affected local populations, on average across all burns over this time period, the severity is likely to be much lower.
Dams & water management/use
The removal, degradation, and fragmentation of foraging and roosting habitat (e.g., forests, wetlands and riparian areas) can be caused by a variety of anthropogenic sources, including dams and the associated changes in water flow patterns. Large areas of bat habitat may be deforested and flooded during dam construction and activation; any hibernacula in the flood zone will become submerged and unusable. Changes to water flow patterns could exacerbate, or accelerate blockages of airflow, create changes to the hydrology or microclimatic conditions within hibernacula, cause flooding, or directly degrade the habitat (McAlpine 1983, U.S. Fish and Wildlife Service 2007). A study in Europe found a decline in bat activity over the area submerged by a large dam construction project while activity increased in the area surrounding the reservoir (suggesting displacement) (Rebelo and Rainho 2009).
At least two new large dams are anticipated in the next ten years, which could impact bat habitat. Flooding in winter could potentially kill bats in hibernacula, while summer flooding could potentially impact maternity roosts. Overall, these projects are likely to impact a negligible proportion of the total population (based on known projects), but within these areas, severity could be extreme for large projects, though much lower for small projects. Averaged across affected populations, a severity of serious to extreme is expected.
4.2.8 Invasive & other problematic species & genes
Invasive non-native/alien species – White-nose Syndrome
White-nose syndrome (WNS), caused by the dermatophyte
Pseudogymnoascus destructans is found widely throughout Europe and the Palearctic, including in Northeastern China (Martínková et al. 2010, Wibbelt et al. 2010, Puechmaille et al. 2011, Hoyt et al. 2016, Zukal et al. 2016). The North American strain of the fungus is believed to have originated in Europe (Lindner et al. 2011, Pikula et al. 2012, Ren et al. 2012, Warnecke et al. 2012, Leopardi et al. 2015) and was first detected in the U.S. in 2006 (Lorch et al. 2011) and in Canada in 2010 (U.S. Fish and Wildlife Service 2018). The fungus grows in the same microclimate conditions that occur within hibernacula where the three species of bats overwinter. Once introduced into a bat hibernaculum, P. destructans is capable of environmental growth, without requiring the presence of bats; consequently it is believed that the fungus can remain viable in abandoned hibernacula for decades (Reynolds et al. 2015, Ballmann et al. 2017). Moreover the spores of P. destructans can remain viable in a wide range of environmental conditions for long periods of time (Hoyt et al. 2015b). The ability of the spores to remain viable in the soil, guano and on the walls of hibernacula may potentially impede recovery of these species (Langwig et al. 2012, Hoyt et al. 2014).
The fungus colonizes the bat’s skin, causes erosions of the epidermis, and damages sweat glands, oil-producing glands, muscles, connective tissue, blood vessels, and hair follicles (Meteyer et al. 2009, Cryan et al. 2010). The wings and ears develop white-grey blotches on their surfaces and the muzzle often turns fuzzy white.
The early stages of WNS may not be visible in all affected individuals. WNS is characterized by an elevated metabolic rate associated with the epidermal fungal growth, stimulating hyperventilation, and results in increased arousals from torpor that contribute to dehydration and electrolyte loss (Warnecke et al. 2012, Warnecke et al. 2013, Verant et al. 2014). Later stages of WNS are associated with more extensive and severe tissue lesions, further increasing arousal frequency, water loss, and energy use (Warnecke et al. 2012, Warnecke et al. 2013, Verant et al. 2014). Energy reserve depletion is accelerated by reduced torpor bout lengths and through acute physiological changes as WNS pathology progresses, eventually leading to mortality (Frank et al. 2014, Verant et al. 2014). WNS-infected bats are more likely to fly (and fly erratically) during winter (Carr et al. 2014). Bats that survive until spring may have damaged wings with numerous holes, and may exhibit signs of physiological stress, and reduced reproductive success (Reeder and Turner 2008, Meteyer et al. 2009, Reichard and Kunz 2009, Powers et al. 2012). However, almost all of the identified mortality associated with WNS has been during hibernation when the immune functions of bats are reduced (Cryan et al. 2010). Additionally, Fuller et al. (2011) tracked individual Little Brown Myotis and found wing damage caused by WNS healed to some degree throughout the summer. Prevalence decreases during the summer months, likely as a result of body temperatures above that required for P. destructans (Langwig et al. 2015b).
In eastern Canada and the northeastern United States, WNS mortality rates are typically low (i.e., 20%) in the first year of detection, followed by high levels (i.e., >70%) within two years (Frick et al. 2010a). At known hibernacula in eastern Canada, the number of hibernating Little Brown Myotis and Northern Myotis bats has declined by an estimated 94% (COSEWIC 2013). The number of Tri-colored Bats has also shown a precipitous decline of approximately 75% at known hibernacula (Turner et al. 2011, COSEWIC 2013).
Rate of spread
From the epicenter in Albany, New York, the rate of spread of WNS in Canada has been approximately 200 to 250 km per year (COSEWIC 2013). As of 29 May 2018, WNS was recorded in 32 states and 7 provinces; the presence of P. destructans was confirmed in 2 additional states (U.S. Fish and Wildlife Service 2018) (Figure 1; Table 1). This represents approximately 21% of the Canadian range of Little Brown Myotis, 30% of Northern Myotis and 100% of Tri-colored Bat that have been impacted by WNS (Figures 1-3). Additional sites are being detected each year in Canada, with the most recent (i.e., 2018) western detection of P. destructans in Manitoba, in the Lake St. George area (U.S. Fish and Wildlife Service 2018). WNS was confirmed in Washington State in March 2016, approximately 150 km from the Canadian border, and represents an approximately 2100 km jump from the previously-known westernmost detection of the fungus (U.S. Fish and Wildlife Service 2018). It is possible that the fungus went unnoticed for several years and suggests caution in defining areas as not yet affected by WNS.
It is uncertain if WNS has spread to Labrador, as well as the northern-most parts of Ontario and Quebec because there is limited information related to the location and characteristics of hibernacula in these regions. To date, testing of a few known hibernacula on the island of Newfoundland has not been positive for Pd though WNS has been confirmed in three individual Little Brown Myotis in western Newfoundland (S. Pardy-Moores, pers. comm. 2018). The Côte-Nord region of Quebec is also currently believed to be WNS-free.
Transmission
Transmission occurs as a result of bat-to-bat contact and contact with contaminated hibernacula, as well as human-assisted mechanisms (e.g., tourists, spelunkers, and researchers that do not follow proper decontamination protocols) (Lorch et al. 2011, Lorch et al. 2013). While it is believed that the spores are spread primarily from bat to bat, the role of environmental factors in its dispersal is not yet well understood. (Puechmaille et al. 2011, Lorch et al. 2013). The amount of physical contact among hibernating bats varies by species, and does not correlate well with infection rates (Kilpatrick 2013, Langwig et al. 2015b). Transmission of P. destructans does not appear to be associated with winter colony sizes or influx of susceptible individuals after the mating season (Langwig et al. 2015b). Until there is a better understanding of the relationship of the environmental persistence of P. destructans and the dynamics behind its spread, factors which exacerbate its spread should be addressed, specifically human activities which could be contributing to its distribution (Shelley et al. 2013).
Accumulations of guano in caves have been identified as being a potentially permanent reservoir for the fungus causing WNS (Mulec et al. 2013, Raudabaugh and Miller 2013, Reynolds and Barton 2014). Bat guano is used as a fertilizer for private gardens and for the commercial production of certain field crops, and it is largely sourced from bat hibernacula in Asia and Europe. Several protocols are being developed for the decontamination of surfaces; however, there is no known regime to sterilize P. destructans spores in bat guano. The movement and distribution of bat guano from eastern to western North America could hasten the spread of White-nose Syndrome, as could its importation from Europe or Asia.
Once bat guano containing P. destructans spores has been used as a fertilizer, the spores may be transported from a garden or field to bats or their hibernacula through the movements of predators such as raccoons, which take advantage of congregations of bats (Fenton and Barclay 1980a, McAlpine et al. 2011). After the arrival of WNS, McAlpine et al. estimated that at one cave in eastern Canada, raccoons consumed thousands of dead and dying Little Brown Myotis and Northern Myotis. They postulated that raccoons foraging in multiple hibernacula may contribute to the dispersal of P. destructans spores (McAlpine et al. 2011) as could a person using the same foot wear in a garden and subsequently visiting a hibernaculum. Ectoparasites have been proposed as assisting in the transmission of the spores from bat to bat (Lučan et al. 2016); other insects, such as lepidopterans are also possible vectors.
Infection of the remaining Canadian range of Little Brown Myotis and Northern Myotis
If WNS continues to spread at its current rate, all hibernacula in Canada and the U.S. will be infected by 2025 to 2028 (Maher et al. 2012, COSEWIC 2013, O'Regan et al. 2014). Notwithstanding the 2016 detection of WNS in Washington State, there is much uncertainty as to how WNS will spread in the west and north due largely to the species differing overwintering ecology and possibly due to differences in climate.
In Canada, as WNS approaches less forested regions of southwestern Manitoba, the relative dryness and few trees in the Prairies suggests that the westward transmission of WNS by Northern Myotis may occur at a slower rate. Davy et al. (2015) suggests that the potential spread of the fungus that causes WNS into north-central Canada may be retarded by the opposing direction of gene flow of Little Brown Myotis in central Canada. However, it is possible that WNS may spread westward using alternative routes (e.g., from the south). Moreover, despite some evidence that the Rocky Mountains historically restricted bat gene flow between eastern and western areas, mixing exists and therefore it is unlikely that the mountains will be a physical barrier that prevents WNS from reaching the western coastline through bat-assisted spread (Russell et al. 2012).
WNS may reach WNS-free populations faster than would be expected from bat-to-bat transmission because of human-assisted transmission of the P. destructans spores, as is implied with the 2016 detection of WNS in Washington State. Pseudogymnoascus destructans was probably brought to North America on the clothing of tourists who had visited caves in Europe (Okoniewski et al. 2010). People who visit multiple caves without decontaminating their clothing or gear substantially increase the risk that P. destructans will be transmitted to WNS-free hibernacula. In addition, bats can be inadvertently transported. For example, there are reports of bats being transported to British Columbia within the cargo hold of a ship (P. Govindarajulu, pers. comm.) and in the awnings of camper vans (D. Hobson and G. Horne, pers. comm. 2015). Such incidences have the ability to greatly increase the rate at which WNS spreads.
Resistance and treatment
A small percentage of individuals may have a genetically-based resistance or immunity to the effects of P. destructans that would be passed to their offspring. In Central Europe, P. destructans has been recorded in approximately 63% of sampled hibernacula, and on several bat species, yet mortality due to WNS has not been observed suggesting that populations of European species may be resistant to, or tolerant of, WNS (Wibbelt et al. 2010, Horacek et al. 2012). Reichard et al. (2014) found a small number (113 / 2095 banded individuals) of Little Brown Myotis in New England survived 1 to 6 winters since the arrival of WNS and some showed signs of reproductive success. Researchers in Ontario have also documented small numbers of Little Brown Myotis surviving WNS infection and reproducing (D. Morningstar, pers. comm. 2015).
Substantial research is ongoing into the ultimate causes, treatment, and mitigation of WNS. The major molecular component responsible for the effects of P. destructans has been identified and could represent a target for WNS intervention (O’Donoghue et al. 2015). Promising new research has also isolated an enzyme that naturally occurs on the skin of bats that appears to inhibit the invasion of tissue by P. destructans in laboratory tests (Hoyt et al. 2015a). Additionally, research has demonstrated that substances produced by the soil bacterium Rhodococcus rhodochrous and the yeast Candida albicans may have potential as biological control agents of P. destructans (Cornelison et al. 2014, Raudabaugh and Miller 2015).
The impact of the threat of WNS on bat populations represents the single most important threat to these three species of bats in Canada with population declines that could result in extirpation.
Invasive non-native/alien species – cats
Domestic and feral cats are known to prey upon a substantial number of birds (Calvert et al. 2013), small mammals (Loss et al. 2013), reptiles, and amphibians (Loyd et al. 2013). Ancillotto et al. (2013) suggested that cats may be a significant threat to bats. The Community Bat Programs of British Columbia regularly receives calls and anecdotal reports of cats killing bats in British Columbia (J. Craig, pers. com.). Species that roost within anthropogenic structures (e.g., barns), such as Little Brown Myotis, are likely more susceptible to this threat because of their potential close proximity to cats. A predation event within a maternity colony, particularly while young are not yet capable of flight, has the potential to significantly affect the breeding success of that colony. In Italy, adult female bats in rural or sparsely urban areas were most likely to be preyed upon by cats (Ancillotto et al. 2013). In Yukon, cats tend to kill juvenile Little Brown Myotis that have recently become capable of flying (T. Jung, pers. comm. 2015).
Given the large numbers of bats using urban areas, the scope of bats potentially encountering cats could be restricted to large (11-70%). However, while there may sometimes be severe impacts on individual colonies, average impacts of colonies affected by cats are probably slight (1-10%).
Problematic native species
Increased incidence of seasonal algal blooms may also pose a threat to these species. Algal blooms can be a natural occurrence, however, there appears to be an escalation in the global occurrence of blooms that are harmful or toxic (Hallegraeff 1993, Anderson et al. 2002). Algal blooms occur as a result of increased nutrient inputs to waterbodies; phosphorus and nitrogen from industrial, agricultural and sewage sources are the two most important human-derived inputs (Anderson et al. 2002). These inputs allow for the production of harmful and/or toxic algae, including a chemical called microcystin. Microcystin is known to cause skin irritations, vomiting, cancer of the liver and death in humans, livestock, pets and many aquatic organisms (Kuiper-Goodman et al. 1999, Sivonen and Jones 1999). Researchers have found the transfer of microcystin from aquatic to terrestrial ecosystems, namely through the emergence of aquatic insects (Smith et al. 2008) which in turn are ingested by bats. Microcystin was detected in all fecal samples (n=20) of Little Brown Myotis near a lake in Michigan that experiences seasonal algal blooms (Woller-Skar et al. 2015). Another toxin associated with algal blooms (Anabaena flos-aquae) was implicated in a mass mortality event of bats (including Myotis spp.) in Alberta (Pybus et al. 1986).
Further study is required to understand the impacts to individual bats and populations, however, this toxin may represent a previously unrecognized threat to bat populations (Woller-Skar et al. 2015). Little Brown Myotis may be more vulnerable to this threat because of the way they forage over water, including skimming insects from the water’s surface. Tri-colored Bats also tend to forage over water, though at higher heights than Little Brown Myotis.
However, such blooms are likely to impact a negligible proportion of populations, and impacts on those would likely be negligible, but perhaps could be slight. Northern Myotis tend to forage within and alongside forests and thus their exposure to this threat is negligible.
4.2.9 Pollution
Industrial & military effluents
Reported sub-lethal effects of chemical contaminants in bats include impairment of flight and foraging ability, resulting in higher predation risk and lower energy accumulation, immunosuppression, reduced reproductive success, and change in metabolic activity (Clark and Lamont 1976, Eidels et al. 2007, Kannan et al. 2010). The effect of sub-lethal contamination on the resulting susceptibility of bats to WNS is still unclear, but high concentration of organic contaminants in Little Brown Myotis fat tissues were found both in healthy populations and populations affected by WNS (Kannan et al. 2010).
Oil spills, tailing ponds and effluent from sources such as mining and pulp and paper production can contaminate the aquatic habitats that bats rely on for their insect prey. Waste rock and mine tailings can result in release of chemical contaminants to both water and soil; acidic draining and the leaching of metals may also occur (Environment and Climate Change Canada 2015). Polychlorinated biphenyls (PCBs) are industrial chemicals which are very persistent both in the environment and in living tissue. The most obvious signs of environmental harm caused by PCBs are in aquatic ecosystems and in species that eat primarily aquatic organisms (Environment and Climate Change Canada 2015).
Little Brown Myotis and Tri-colored Bat primarily feed on flying and emerging aquatic insects over water while Northern Myotis primarily glean terrestrial insect prey within and along forests. This would suggest a greater susceptibility of exposure to the contaminants for the former two species. Irrespective of the species, there is no evidence that industrial effluents are regularly encountered by most bat populations. There is no published evidence of population level impacts on bats and impacts, even in affected areas, are likely to be slight to negligible.
Agricultural & forestry effluents
Pesticide spraying in agricultural or forested landscapes has the potential to reduce the abundance of insects on which bats feed and has the potential to affect bats through the ingestion of contaminated prey. Widespread and/or continuous application of pesticides (such as that which might occur for Spruce Budworm - Choristoneura fumiferana, Mountain Pine Beetle - Dendroctonus ponderosae, or on agricultural landscapes) could potentially have substantial impacts on food availability and physiology. Even at local scales in the United Kingdom, bat activity was significantly higher in aquatic habitats of organic farms versus conventional farms, suggesting greater prey availability in areas with lower levels of agrochemical use (Wickramasinghe et al. 2003).
Neonicotinoid insecticides were introduced in the 1990s, are currently the most widely used class of insecticides globally, and their use is continuing to increase (Sparks 2013, Douglas and Tooker 2015). They are generally used on agricultural lands, but have been detected in wetlands (Main et al. 2014) and watercourses in Canada (Environment Canada 2011, Xing et al. 2013) and are frequently found at levels that exceed water quality guidelines (Morrissey et al. 2015). Neonicotinoids adversely affect insect populations (and therefore potential prey of bats) (Goulson 2013); some of the most important prey (flies, caddisflies, and mayflies) of Little Brown Myotis, Northern Myotis, and Tri-colored Bats are among the most sensitive aquatic insects to neonicotinoids (Morrissey et al. 2015). Reduced prey availability could potentially result in increased time spent foraging, less fat stores and/or increased time spent in torpor, ultimately leading to poor body condition and resulting in reduced reproductive and/or survival rates (Talerico 2008, Reimer 2013). In addition to reduced prey populations, neonicotinoids also cause direct sub-lethal effects on the reproductive success, development, immune function, and growth in numerous vertebrates (Gibbons et al. 2015). Mason et al. (2013) hypothesized that the thousands of invertebrates consumed by bats would inevitably expose bats to small cumulative doses of these toxins. To date, no research has explored the direct or indirect effects of neonicotinoids on bats.
Insecticides are only applied to a small percentage of Canada's total agricultural and forestry area each year, often in the same areas in multiple years (Statistics Canada 2018) but given that effects of insecticides may expand beyond where they are applied, especially if they enter water supplies, the scope is considered to be large. However, given that bat populations are already greatly reduced due to White-nose Syndrome, it seems unlikely that declines in insects, even in heavily affected areas, would be large enough to cause food shortages for bats leading to further declines of more than 30% over next 15-30 years, and average impacts are likely much lower. There is no evidence of population level impacts on bats related to direct mortality or impacts on reproduction from pesticides (except if deliberately used to kill bats, which is covered in threat 5.1). Agricultural effluents that cause toxic algal blooms from increased nutrient loads in wetlands/waterbodies, and mercury and acid rain are captured elsewhere.
Air-borne pollutants
Mercury is a naturally occurring element that is enriched in the environment by human activities. Long-range atmospheric transport and deposition is the dominant source of mercury to many aquatic habitats over much of the landscape (Fitzgerald et al. 1998, U.S. Geological Survey 2000). Bio-available mercury is also mobilized within watersheds by forestry activities, hydroelectric reservoir creation, and various industrial-related activities (Porvari et al. 2003, Vuori et al. 2003, Wiener et al. 2003). Mercury concentrations in aquatic food webs are usually correlated with low pH levels, and as a result mercury concentrations increase from west to east across Canada, along with pH levels, in freshwater food webs (Depew et al. 2013).
Bat species appear to be particularly susceptible to heavy metal accumulation because most species are long-lived, occupy high trophic levels, feed on aquatic emergent insects, and sustain high metabolic rates and food intake. A study in the northeastern United States that collected samples of ten different bat species from point-source and non-point source locations found the highest concentrations of mercury in Tri-colored Bat, Little Brown Myotis and Northern Myotis (Yates et al. 2014). This study also found significantly higher accumulation of mercury in blood and fur samples in Little Brown Myotis and Northern Myotis in all age classes near WNS contaminated sites than in non-contaminated locations (Karouna-Renier et al. 2014, Yates et al. 2014). However, Karouna-Renier et al. (2014) did not find significant differences in the genotoxic effects (i.e., DNA damage) of mercury between contaminated and non-contaminated sites. Mercury concentrations in Little Brown Myotis sampled across Nova Scotia varied among colonies in relation to nearby lake acidity, and 48% of the individuals sampled had concentrations in excess of a threshold associated with neurochemical changes in other bat species from Virginia (Little et al. 2015b). Of 344 Little Brown Myotis individuals sampled from maternity roosts across Atlantic Canada (Nova Scotia, Prince Edward Island, and Newfoundland and Labrador), 37% had concentrations exceeding the neurochemical threshold (Little et al. 2015a). These recent studies raise concerns regarding the effects of mercury and other environmental contaminants on reproductive success, physiological responses (e.g., immune system responses), and survival.
Because mercury contaminates aquatic systems, it would presumably be of particular concern for both Little Brown Myotis and Tri-colored Bat that feed primarily over water on aquatic insects. However, it has been found that Northern Myotis can also be exposed to significant levels of mercury (Yates et al. 2014). Given that these air-borne pollutants are deposited through long-range atmospheric transport, the scope is pervasive. However, there is no evidence to support population-level impacts so severity is considered negligible to slight.
Excess energy
The alteration (e.g., timing, spatial extent, and spectral signature) of natural light regimes from artificial light sources can impact species in various direct and indirect ways related to foraging, reproduction, communication, habitat use, and movement behavior (Stone et al. 2009, Gaston et al. 2013, Mathews et al. 2015). Impacts may be beneficial (e.g., increased foraging opportunities), neutral, or detrimental (e.g., increased susceptibility to predation, collisions with lighted structures) (Kyba et al. 2011).
For bats in particular, when insect prey becomes concentrated around light sources, foraging efficiency can increase which has been viewed as beneficial to some species (Entwistle et al. 2001, Lacoeuilhe et al. 2014, Mathews et al. 2015). In southwestern Ontario, Furlonger (1987) found all species of bats encountered exploited concentrations of insects around artificial light sources though this was not significant for Myotis spp.; Tri-colored Bats were not encountered. In general, it has been found that bats with high or medium wing-loading and fast flight exploit insects at street lamps while gleaners and flutter-detectors
Though seemingly beneficial to some species of bats, light pollution has been attributed to reductions in many insect populations that are attracted to lights, including moths, aquatic insects, and other terrestrial insects (Frank 1988, Perkin et al. 2014, MacGregor et al. 2015). Many insects are attracted to artificial lights, affecting their dispersal and navigation as well as reproduction, mating, crypsis, and ability to evade predators. Individuals experience direct mortality from flying to exhaustion, burning to death, or becoming trapped in light receptacles (Frank 1988, Horváth et al. 2009, Perkin et al. 2014). This in turn reduces the biomass and abundance, and can change the relative composition of insect populations, creating implications in the food chain through disruption of predator-prey relationships, pollination services, and ecosystem function (Hölker et al. 2010, Kyba et al. 2011).
In Hungary, illumination of the roosts of house-dwelling Myotis species resulted in the collapse of entire colonies and reduced growth rates of juveniles (Boldogh et al. 2007). This was due to delayed emergence from roosts resulting in missed opportunities for foraging during peak insect activity; presumably, the avoidance of lighted areas is due to increased predation risk and/or negative effects on the bats’ orientation ability (Boldogh et al. 2007, Lacoeuilhe et al. 2014).
The impacts of artificial light appear to be variable and species-specific. Little direct research dedicated to understanding the effects of light pollution has been done on these three species of bats in Canada and further study is required.
Mining exploration activities, forestry operations, or other industrial activities may threaten bats if the activities cause noise, light, and vibrations near hibernacula that disturb hibernating bats and cause them to arouse from torpor. Similarly, noise and vibrations in areas where maternity colonies are found may result in reduced reproductive success, roost abandonment, and relocation to other sites (McCracken 2011). Since bats rely on echolocation or prey-generated sounds to forage, anthropogenic noise could also interfere with foraging and affect prey detection (Bunkley et al. 2015). However, a study of compressor stations associated with natural gas extraction in New Mexico revealed no significant difference in the activity level of Little Brown Myotis at loud compressor sites compared to quieter well pads (Bunkley et al. 2015).
Overall, in the course of an annual cycle, most bats in Canada encounter artificial light or noise.
4.2.10 Climate change & severe weather
The effects of climate change on bats are unknown. Bats (particularly lactating females) are more susceptible to evaporative water loss than other mammals, suggesting that they may be vulnerable to increased temperatures associated with climate change (Webb et al. 1995, Chruszcz and Barclay 2002, Adams and Hayes 2008). Adams (2010) found significant reproductive declines in Little Brown Myotis in years that mimicked predicted conditions related to future climate change scenarios (i.e., reduced availability of water) for western North America.
Other direct effects include the destruction of roosts and/or hibernacula as a result of increased storm frequency that is predicted to occur in the future (Jones and Rebelo 2013). Although warmer temperatures as a result of climate change could benefit hibernating bats in Canada, it may also lead to a disruption of hibernation, a reduction in water, and increased disease (Sherwin et al. 2012). Humphries et al. (2002) predicted climate change to cause a northward range expansion of Little Brown Myotis within 80 years.
The extent, intensity, and frequency of forest fires are projected to further increase because of warmer and drier springs and summers (Flannigan et al. 2009, de Groot et al. 2013, Girardin et al. 2013). Similarly, forest insect outbreaks (e.g., Spruce Budworm, and Mountain Pine Beetle) may intensify with the changing climate (Mattson and Haack 1987). These processes have the ability to alter large forested areas and cause whole tree mortality (Fleming et al. 2002), but may also create available snags for bat roosting and/or increase local prey availability (Wilson and Barclay 2006). Therefore, the ultimate impacts on bat populations and their habitats are unknown.
In addition to direct effects, climate change is predicted to indirectly affect bat survival through its effect on insect populations (Arlettaz et al. 2001). In northeastern regions of the continent, climate change is expected to cause wetter winters and drier summers (Hayhoe et al. 2007, Huntington et al. 2009). Adult female Little Brown Myotis have reduced annual survival in dry years, presumably related to the link between moisture availability and emergent insect availability (Frick et al. 2010b). The timing of peak abundances in some insects have also become earlier (Both et al. 2009). This may affect the synchronicity of peak prey densities and bat breeding (Jones et al. 2009) and therefore pup survival; sufficient prey is needed for pups to gain the fat tissue that is necessary for overwinter survival (Kunz et al. 1998).
5 Population and distribution objectives
There are no reliable current or past population estimates for Little Brown Myotis, Northern Myotis, or Tri-colored Bat in Canada. As such, population trends will be estimated using data from known and previously surveyed hibernacula and roosts throughout the species’ Canadian range (as presented in COSEWIC 2013) as an index of the total population trends, and augmented by surveys and monitoring presented in section 6.2: Strategic Direction for Recovery of this recovery strategy.
The population and distribution objectives for Little Brown Myotis and Northern Myotis are presented together due to the similarities among the two species, such as belonging to the same Genus, having large geographical distributions extending across much of Canada and exhibiting similar declines in response to WNS. Tri-colored Bat is presented separately due to it belonging to a different Genus and its smaller distribution that has been entirely impacted by WNS.
A short-term and long-term population objective is presented for all three species within areas known to be affected by WNS. The short-term objectives provide the benchmarks to achieving the long-term objectives and provide an opportunity to review and re-assess along the way. Short-term objectives are presented because it is recognized that the long-term population objectives will take many years to achieve and there is uncertainty as to whether they can be achieved given there are as yet no known techniques to treat WNS or arrest it’s transmission. The long-term objectives represent the desired state of the population in areas affected by WNS and recognize the slow population growth rates of these species.
Little Brown Myotis and Northern Myotis
The observed declines in these two species in areas affected by WNS have resulted in populations whose status and condition differ greatly from populations in areas not yet known to be affected by WNS. Therefore, area-specific population objectives for these two species are defined based on the current status of WNS across the country: WNS-affected areas and areas that are not yet known to be affected by WNS. The areas to which the population objectives apply are considered dynamic in response to the on-going spread of WNS, as the status of WNS is expected to change over time. To obtain an up-to-date map of the current status of WNS refer to the online map made available by the Canadian Wildlife Health Cooperative
Distribution objective:
- The distribution objective for both the Little Brown Myotis and Northern Myotis is to maintain (or where applicable restore to) the pre-WNS extent of occurrence (the area that encompasses the known geographic distribution of the species in Canada as depicted in Figures 1 and 2)
Population objective (within WNS-affected areas):
- Within WNS-affected areas, the short-term (12-18 years) population objective is to stop the declining population trend, or if feasible, achieve an increasing population trend
footnote 17 - Within WNS-affected areas, the long-term (many generations) population objective is a self-sustaining
footnote 18 , resilientfootnote 19 , redundantfootnote 20 and representativefootnote 21 population
The range of WNS overlaps with approximately 28% and 17% of Little Brown Myotis and Northern Myotis’ Canadian ranges, respectively, and is expanding at an average rate of 200 to 250 kilometers per year (COSEWIC 2013). Since conditions suitable for P. destructans growth exist in areas not yet affected by WNS, without mitigation, the entire Canadian population of both species could be affected by 2025 to 2028 (COSEWIC 2013), and perhaps sooner with the 2016 detection of WNS in Washington State. A 12-18 year timeframe was deemed appropriate for the short-term population objective as it represents the timeframe for when the entire range of these two species is predicted to be impacted by WNS.
While declines in both these species have been drastic following the introduction of WNS, there is evidence of an increasing trend in annual survival of Little Brown Myotis post-WNS from a site in New Jersey (Maslo et al. 2015) to support the possibility of achieving the population objective in WNS-affected areas in the short-term. This finding suggests that stabilization of remnant colonies post-WNS are due to increased annual survival rates rather than other factors, such as immigration. However, despite improved annual survival rates, population models based on this study predict continuing population declines in Little Brown Myotis (Maslo et al. 2015). Improving adult and juvenile survival, such as reducing adult and juvenile additive mortality (from all sources, including WNS), is the most important element to target to promote recovery potential in areas impacted by WNS (Maslo et al. 2015).
The long-term population objective in WNS-affected areas is based on the slow population growth rate of these two species, which implies that populations would require many generations (i.e., hundreds of years) to recover (see Limiting Factors section). The degree to which the Canadian (and continental) populations of Little Brown Myotis and Northern Myotis will ever be able to fully recover to their historical levels in known WNS-affected areas is uncertain. The short-term objective recognizes that achieving an increasing population trend (if feasible) will improve the recovery potential of the populations (e.g., ability to re-populate areas affected by WNS and increase the likelihood of finding individuals with resistance to WNS) and, hence, the ability to meet the long-term objectives.
Population objective (areas not yet affected by WNS):
- Within areas not yet affected by WNS, the population objective is to maintain a stable population trend, or if feasible, achieve an increasing population trend
Preventing the introduction of WNS to hibernacula in areas not yet affected (e.g., Prairies, northern Canada, western Canada) is the most important factor for preventing further loss of individuals. In Canada, as WNS approaches less forested regions of southeastern Manitoba, the lower density of trees suggests that westward transmission of WNS by Northern Myotis (that rely on forested areas) may occur at a slower rate. Davy et al. (2015) suggests that the potential spread of the fungus that causes WNS into north-central Canada may be retarded by the opposing direction of gene flow of Little Brown Myotis in central Canada.
Though information on bat population sizes and trends in Canada is lacking, it is believed that populations outside of areas affected by WNS are generally stable and/or increasing. For instance, in the northeastern U.S., Langwig et al. (2012) estimated that bat populations (all species) in general, prior to the arrival of WNS, were growing at an average rate of 8% per year and population trend analyses of hibernacula data from across the U.S. indicated the population trends of these two species of bat in particular were relatively stable (Ellison et al. 2003, Frick et al. 2010a, Frick et al. 2010b). On this premise, the population objective is set at maintaining stable population trends, or improving them (if feasible), As in WNS-affected areas, the objective recognizes that achieving an increasing population trend (if feasible) will improve the species’ recovery potential. Nevertheless, it is acknowledged that it may not be possible to prevent the spread of WNS.
Tri-colored Bat
Distribution objective:
- The distribution objective for Tri-colored Bat is to restore (then maintain) the pre-WNS extent of occurrence (the area that encompasses the known geographic distribution of the species in Canada as depicted in Figure 3)
Short-term (10 years) population objective:
- The short-term population objective is to stop the declining population trend, or if feasible, achieve an increasing population trend
footnote 22 over the next 10 years
The entire range of Tri-colored Bat in Canada has been impacted by WNS. It is expected that extensive research into treatments for WNS on individuals or in hibernacula will be explored. Though post-WNS survival rates are unknown for Tri‑colored Bats, approaches similar to those suggested for Little Brown Myotis and Northern Myotis to improve annual survival of adults and juveniles (or reduce mortality of these demographic groups) are expected to support achieving the population and distribution objectives. Given the entire range of the species is currently impacted by WNS, the 10-year time frame was deemed appropriate for the short-term population objective because determining if the population has stopped declining or is increasing will take multiple years of data acquisition. Furthermore, the COSEWIC criteria for assessment include reviewing population change within 10-year windows.
Long-term population objective:
- The long-term population objective is a self-sustaining, resilient, redundant and representative population
The degree to which the Tri-colored Bat population will be able to fully recover to its historical levels is uncertain. The long-term population objective is based on the expectation that, even if individuals develop resistance or a treatment for WNS is found, the slow population growth rate of this species means populations would require many generations (i.e., hundreds of years) to recover (see Limiting Factors section).
Because the Canadian population of Tri-colored Bat occurs at the northeastern part of its continental range, and the vast majority of its population and distribution occurs in the United States to the eastern coast of Central America, population changes at the continental level will have a significant effect on recovery feasibility in Canada.
These objectives may be revised as new information related to WNS and bat populations across Canada become available.
6 Broad strategies and general approaches to meet objectives
6.1 Actions already completed or currently underway
The following list of actions is not exhaustive, but is meant to illustrate the main areas where work is already underway and to give context to the broad strategies to recovery outlined in section 6.2. Actions completed or underway include the following:
International:
- In April 2015, a ‘Letter of Intent Related to Efforts to Promote Conservation of Bats in the United Mexican States, the United States of America and Canada’ was signed by representatives of each of the three countries to increase collaboration and coordination of bat conservation across North America
- The North American Bat Monitoring Program (NABat) is creating a continental-wide coordinated effort for monitoring bats at local to range-wide scales to provide reliable data that will promote effective conservation decision-making. A Plan for the North American Bat Monitoring Program was released in June 2015 (Loeb et al. 2015)
- North American Bat Conservation Alliance (NABCA) facilitates communication between bat organizations across North America, develops conservation priorities, and addresses conservation issues
- The Northeast Bat Working Group and Western Bat Working Group enable information exchange between agencies, organizations, industry, and individuals interested in bat research, management, and conservation, and facilitates multi-state collaborations (Northeast Bat Working Group 2015, Western Bat Working Group 2015)
- The North American Society for Bat Research promotes and develops research of bats and coordinates an annual Northern American Symposium on Bat Research (NASBR 2015)
- The U.S. Fish and Wildlife Service provides funding for research and coordinates an annual WNS workshop or conference that brings together international researchers to present new results and develop management strategies
- There is Canadian representation on the various United States technical working groups involved with WNS response to ensure approaches developed in Canada are consistent with international efforts and that the data collected is able to be shared and compared
National:
- Canadian Wildlife Health Cooperative (CWHC) works in collaboration with federal, provincial and territorial, academic, and non-governmental organizations to organize surveillance for WNS and the fungus that causes it, ensure appropriate standardized diagnostic testing and reporting of surveillance results, develop national decontamination protocols and product assessment, coordinate national-level monitoring of bats, and identify data gaps and data management needs in Canada. CWHC leads Canada’s Inter-agency White Nose Syndrome Committee which contains five technical working groups. This group updated A National Plan to Manage White Nose Syndrome in Bats in Canada in February 2015 and will likely continue to serve as one of the main avenues for national implementation efforts related to WNS (Canadian Wildlife Health Cooperative 2015a)
- Efforts are underway by CWHC, NABCA, and Environment and Climate Change Canada to expand the Edubat project into Canada to increase bat education and outreach (BatsLive 2015)
- The Ministère des Forêts, de la Faune et des Parcs of Quebec in collaboration with the Centre de la science de la biodiversité du Quebec (CSBQ) and the University of Winnipeg developed a website (English; French) to promote a citizen science project for maternity roost monitoring in central and eastern Canada (Quebec, Ontario and Manitoba) and may be expanded to other parts of the country. The website presents documentation about bats, their conservation, and BMPs for bats in buildings. The website also hosts a maternity roost database, where citizens can enter a roost and its annual counts
- Federally, provincially and territorially, bats are considered for land-use development project screening and permitting and during environmental assessments across Canada. Mitigations measures and pre- and post-development monitoring programs are established as necessary to minimize and evaluate adverse effects
- Environment and Climate Change Canada is developing beneficial management practices (BMPs) for the forestry, wind energy, mining, and nuisance wildlife control industries, as well as for bats in buildings
- Researchers at University of Winnipeg and Trent University are investigating the genetic response of bats pre- and post-WNS across Canada
- The Canadian Wildlife Federation has several outreach activities related to bats (Canadian Wildlife Federation 2015). For example, they have a national bat box program used to distribute bat boxes and encourage citizens to track occupancy
- Several national parks across Canada are conducting bat monitoring using a national protocol developed by Parks Canada Agency and contributing to NABat
- Parks Canada Agency has produced a video with support from CWHC to illustrate the use of decontamination protocols, along with information related to bats and WNS
- Under the National Park General Regulations, caves within Canada’s national park system are closed unless specifically permitted by a park superintendent
- Parks Canada Agency has prepared internal national standards and best management practices for bats using hibernacula and for maternity roosts on PCA managed lands
- Parks Canada has prepared numerous bat outreach and education materials (videos, games, Qs and As, etc.) to provide Canadians with information about bat conservation and encourage all to take action for their recovery
Western and northern Canada:
- The Western Canada Bat Network (WCBN) facilitates information exchange between groups and individuals involved in bat research, management, and conservation in western Canada, Alaska, and some northwestern states, primarily through semi-annual newsletters
- In British Columbia, the Community Bat Programs of British Columbia promotes conservation of bats on private lands, provides a resource to landowners dealing with bat issues, and engages citizen scientists to collect data on bat populations. They also conduct roost emergence counts at maternity colonies to establish baseline relative abundance data, against which future declines can be measured (Community Bat Programs of BC 2014)
- The Community Bat Programs of BC has developed BMPs for pest control techniques and has started outreach initiatives with provincial pest management companies (Community Bat Programs of BC 2014)
- The British Columbia Bat Action Team, in collaboration with the British Columbia Ministry of Environment has published BMPs for caving activities and mining and wind energy industries (Holroyd et al. 2016)
- Winter bat activity is being monitored throughout southern British Columbia by Dr. Cori Lausen (Wildlife Conservation Society Canada), Environment and Climate Change Canada-Canadian Wildlife Service, the British Columbia Ministry of Environment (Dr. Purnima Govindarajulu) and others; winter activity across northern and central British Columbia is also being monitored by Dr. Cori Lausen
- The British Columbia Ministry of Environment and Dr. Cori Lausen have developed appropriate hygiene protocols for bat researchers, cavers and others visiting/working in underground bat habitats
- The British Columbia Ministry of Environment and the Royal British Columbia Museum are archiving carcasses from bat mortality incidents and bat DNA to establish baseline information for British Columbia. A complete health assessment including testing to detect WNS, rabies and other parasites is conducted by Dr. Chelsea Himsworth (British Columbia Ministry of Agriculture) on all the incidental mortality bat carcasses that are recovered
- Dr. Cori Lausen is leading a liaison project (Bats and Cavers Project - BatCaver.org) with the caving community in British Columbia and Alberta to locate caves and mines used by bats
- The British Columbia Ministry of Forests published the Karst Management Handbook for British Columbia (BC Ministry of Forests 2003) to assist forest planners in developing appropriate management practices when conducting forest operations in karst terrain
- Thompson Rivers University, Dr. Ann Cheeptham, funded by US Fish and Wildlife Service is investigating potential sources of fungus-inhibiting microbes from caves, including the fungus that causes WNS
- Alberta Environment and Parks has developed guidelines for minimizing the impacts of wind energy development on bats and protocols for pre- and post-construction surveys
- Alberta Environment and Parks participates in NABat, monitors known hibernacula, searches for new overwintering locations, delivers outreach on bats and has Sensitive Species Protocols that include bats
- The Alberta Bat Action Team develops protocols (e.g., decontamination), identifies research and management priorities, and facilitates information exchange
- Alberta Community Bat program is a new initiative in Alberta to provide resources to private landowners, and engage citizens in bat conservation efforts including locating and reporting roosts
- Saskatchewan’s Environmental Code includes a requirement for any industrial project in the province to ensure it is not impacting bats. The specific provisions pertaining to mines include the requirement of surveys for cave animals and habitat prior to mine reclamation activities
- The Saskatchewan Ministry of Environment is finalizing guidelines for siting, monitoring and operation of wind energy projects in the province
- The Government of Yukon surveys Little Brown Myotis and Northern Myotis to better document their occurrence, reproductive status, and range in Yukon
- The Government of Yukon and Government of the Northwest Territories conduct public education through interpretative events and brochures
- The Government of Yukon engages in various research projects including studying the effects of natural disturbances, the importance of old-growth riparian forest, and long-term monitoring at select maternity colonies
- The Government of the Northwest Territories is gathering baseline information on bats throughout Northwest Territories, searching for undiscovered hibernacula, and conducting WNS surveillance, population monitoring, and management planning at known hibernacula
- Various partners (including Government of the Northwest Territories, Sahtu Renewable Resources Board, industry, academic researchers and community members) have erected acoustic bat recorders at several locations in the Sahtu, Dehcho, South Slave and North Slave regions of the Northwest Territories to learn more about the distribution and activity patterns of bats in these regions
Central Canada:
- Manitoba Conservation and Water Stewardship have taken a number of actions aimed at protecting Little Brown Myotis and Northern Myotis. For example, the locations of hibernacula are considered confidential and visitation to hibernacula is discouraged for any reason other than research. Researchers are required to follow decontamination procedures upon entering and leaving hibernaculum as a condition of their research permit. Some caves used by hibernating bats are protected in an ecological reserve and are gated to prevent human access
- The Ontario Ministry of Natural Resources and Forestry has developed guidelines for minimizing the impacts of wind energy development on bats and is working with the Ministry of Northern Development and Mines and the mining industry to minimize industrial disturbance to bats and their habitats
- Ontario’s Forest Management Guide for Conserving Biodiversity at the Stand and Sites Scales includes direction designed to maintain suitable habitats and habitat features, including hibernacula (Ontario Ministry of Natural Resources 2010)
- The Ontario Ministry of Natural Resources and Forestry has developed Ontario’s White-nose Syndrome Response Plan (Ontario Ministry of Natural Resources and Forestry 2015)
- The Ontario Ministry of Natural Resources and Forestry acoustically monitors bat populations during summer and winter throughout Ontario
- Environment and Climate Change Canada has collected radar and acoustic data in southern Ontario to determine if bats exhibit broad-front migratory movements or concentrate along linear landscape features
- Summer population monitoring is underway in Ontario by the University of Guelph and Myotistar
- Myotistar and Gray Owl Environmental Inc. (with support from Environment and Climate Change Canada and the National Speleological Society) are conducting advanced roost use monitoring at a significant summer roost near Cambridge, Ontario where bats are surviving and reproducing despite being in a WNS endemic region of Canada
Eastern Canada:
- The Ministère des Forêts, de la Faune et des Parcs of Quebec continues to track the spread of WNS in various natural hibernacula during winter and spring. In summer, various initiatives have been put in place to monitor bat populations. The department is conducting acoustic surveys in various regions of Quebec through the acoustic surveillance network of bats (Réseau Chirops). This network, established in 2000 by the Ministère des Forêts, de la Faune et des Parcs of Quebec in collaboration with the Montreal Biodôme and Envirotel, consists of 14 acoustic inventory routes in various Quebec regions. The department also conducts maternity roost counts in anthropogenic structures (Neighbourhood Bat Watch). More intensive monitoring is in place on the North Shore where WNS has not been detected
- A bat recovery team for Quebec was created in 2014. The team is developing a recovery plan for the province and coordinating provincial-level recovery actions
- Bat populations are being monitored regularly by the Department of National Defence at Canadian Forces Base Valcartier
- St. Mary’s University in Nova Scotia and Environment and Climate Change Canada are investigating mercury levels in Little Brown Myotis in Atlantic Canada
- The New Brunswick Museum has monitored the spread of WNS, and associated rate of mortality at known hibernacula throughout the province during pre- and post-WNS years, and continues to provide research in the pervasiveness of the pathogen, the cave microfauna, and environmental conditions
- The New Brunswick Museum, in collaboration with New Brunswick Department of Natural Resources, have established a reporting program to collect observations of winter day-flying bats, leading to new information on the distribution of these species in the province
- New Brunswick Department of Natural Resources has developed survey guidelines for bat and bird mortality during pre- and post-construction periods of wind farm developments in New Brunswick
- Prince Edward Island’s Forest, Fish and Wildlife Division promotes the reporting of bat sightings and works with the CWHC to implement bat monitoring programs
- Nova Scotia Bat Conservation by the Nova Scotia Department of Natural Resources monitors bats during the spring, summer, and fall
- St. Mary’s University is monitoring winter counts at hibernacula in Nova Scotia
- St. Mary’s University is researching demographics and social behavior of Little Brown Myotis in collaboration with Newfoundland and Labrador Department of Environment and Conservation in Newfoundland and Labrador
- The Government of Newfoundland and Labrador continues WNS surveillance and monitors known populations. In collaboration with Memorial University of Newfoundland and Parks Canada, acoustic monitoring takes place to determine species’ presence and abundance. Surveys for Little Brown Myotis and Northern Myotis in Labrador are conducted to better document the occurrence, reproductive status and range
- In the summer of 2015, CWHC was contracted by PEI National Park to acoustically monitor habitat with stationary and mobile detectors. Coastal dunes, forest edges, wetlands, and fresh water sites were monitored, as well as a selection of decommissioned buildings in the national park. Acoustic monitoring will continue for multiple years to analyze bat echolocation activity trends
- Atlantic Coastal Action Plan (ACAP) Cape Breton has been monitoring bats on Cape Breton Island since 2013. Monitoring efforts primarily involve long-term deployment of acoustic detectors in summering habitat and at known and potential hibernacula, and conducting maternity colony counts. In 2015, the program expanded by including additional monitoring sites in New Brunswick, Quebec and Newfoundland though partnerships with La Société d'aménagement de la rivière Madawaska, the New Brunswick Museum, Attention FragÎles and ACAP Humber Arm
6.2 Strategic direction for recovery
The Canadian Wildlife Health Cooperative (Canadian Inter-agency White Nose Syndrome Committee) released a revised version of A National Plan to Manage White Nose Syndrome in Bats in Canada in February 2015 (Canadian Wildlife Health Cooperative 2015c). Whenever possible, the approaches in Table 5 were developed to align with initiatives outlined in that plan. When appropriate, the approaches were categorized into those that are applicable to areas not known to be affected by WNS and those that are applicable to known WNS-affected areas (and the invasion front). The current western invasion front in Canada is in Manitoba, in the Lake St. George area while the eastern invasion front is in western Newfoundland (as of 29 May 2018) (CWHC 2018, U.S. Fish and Wildlife Service 2018).
Threat or Limitation | Broad Strategy to Recovery | Priorityp | General Description of Research and Management Approaches |
---|---|---|---|
Knowledge gaps to recovery | Monitoring and surveys | High | Entire range:
|
Knowledge gaps to recovery | Monitoring and surveys | Medium |
|
Knowledge gaps to recovery | Monitoring and surveys | Low |
|
Knowledge gaps to recovery and all threats | Research | High | Known WNS-affected areas (and invasion front):
|
Knowledge gaps to recovery and all threats | Research | Medium | Entire range:
|
Knowledge gaps to recovery and all threats | Research | Low |
|
Knowledge gaps to recovery and all threats | Education and awareness, partnerships and stewardship | High |
|
All threats | Habitat and species conservation and management | High | Entire range:
|
All threats | Habitat and species conservation and management | Medium | Entire range:
|
All threats | Law and policy | High | Entire range:
|
p “Priority” reflects the degree to which the broad strategy contributes directly to the recovery of the species or is an essential precursor to an approach that contributes to the recovery of the species.
6.3 Narrative to support the recovery planning table
Recovery of Little Brown Myotis, Northern Myotis, and Tri-colored Bat will require commitment, resources, collaboration, and cooperation among federal, provincial, and territorial jurisdictions, wildlife management boards, Aboriginal people, species’ experts, researchers, local communities, landowners, industry, and other interested parties from Canada and the United States. Because of their widespread range across the country, it will be important to apply adaptive landscape-scale management both in the response to the threat of WNS and in the management of bat habitat. For the purposes of evaluating the effectiveness of recovery efforts, and adjusting them where necessary, it is also important to monitor the spread of WNS, hibernacula, maternity roosts, population trends, and the distributions of the three species of bats.
A comprehensive approach to research and monitoring (which includes all stages of the annual life cycles and the entire extent of occurrence) will be required to more completely understand the status, limiting factors, significant threats, and habitat use of each species. Currently, there are no reliable population estimates for any of the three bat species and, in the short-term, it is not realistic to expect that population estimates will be derived. To determine the success of conservation and management efforts, and determine if the population and distribution objectives have been met, an effective monitoring program is needed to measure relative changes in bat abundance over time. To maintain a uniform sampling method across Canada, the protocol of the North American Bat Monitoring Program (NABat) should be used, to the extent possible, as the Canadian national sampling framework (Loeb et al. 2015).
Because WNS is spreading quickly across Canada, research and monitoring pertinent to the fungus must be performed promptly and efficiently before opportunities are lost. This will likely require a large body of researchers, governments, industry, Aboriginal communities, and volunteers to coordinate efforts, communicate effectively, pool resources, and share findings efficiently (Langwig et al. 2015a). Whenever possible, research efforts and priorities should be coordinated through established groups (e.g., North American Bat Conservation Alliance, and CWHC) to avoid duplicated effort and resources. To succeed in preventing the spread of WNS (see section 5: Population and Distribution Objectives), research aimed at a treatment for individuals and/or hibernacula infected with WNS or methods to significantly reduce or prevent the spread of P. destructans will continue to be required.
Education, awareness, partnerships and stewardship are required for reducing risks associated with the human-assisted spread of WNS and to develop and implement initiatives aimed at conserving bat habitats and mitigating other threats. The development of additional beneficial management practices, and the implementation and promotion of existing ones, can assist in managing known threats to the species. Building on existing partnerships and continuing to identify opportunities for collaboration will help to support recovery of bat population both nationally and internationally.
As noted in the threats section (section 4: Threats), in areas where bat populations have significantly declined as a result of WNS, it is important to note that mortality of even a small number of the remaining individuals (particularly juveniles and adults) has the ability to impact the survival of local populations and their recovery. Identifying the importance and risk factors associated with these threats on each of the three species becomes increasingly important (see Appendix B), so that appropriate mitigation measures can be adopted in a timely manner.
While necessary monitoring and research occurs across North America, the current state of available science can provide a base of knowledge to conserve known habitats and mitigate threats to the species. Various beneficial management practices are available for industry and private landowners.
7 Critical habitat
Critical habitat is the habitat that is necessary for the survival or recovery of the species. Section 41(1)(c) of SARA requires that the recovery strategy include an identification of the species’ critical habitat, to the extent possible, as well as examples of activities that are likely to result in its destruction.
7.1 Identification of the species’ critical habitat
The critical habitat identified in this document is considered a partial identification, insufficient to meet the population and distribution objectives. A schedule of studies has been developed to provide the information necessary to complete the identification of critical habitat (see section 7.2: Schedule of Studies). In this recovery strategy critical habitat is partially identified for hibernacula, based on the best available information for each species as of May 2018.
Hibernacula
Hibernacula are used by Little Brown Myotis, Northern Myotis, and Tri-colored Bat to survive when ambient temperatures decline and insects are unavailable, and as such are necessary for the survival and recovery of these species. The availability of suitable hibernacula may be limiting for these species (Ontario Ministry of Natural Resources 2010, COSEWIC 2013).
Occupancy
- Any site where hibernation by Little Brown Myotis, Northern Myotis and/or Tri-colored Bat has been observed at least once between 1995 and 2018 is identified as critical habitat
As a result of human safety concerns, efforts to minimize bat disturbance, and the difficulty in locating and identifying hibernating bat species within complex underground structures, the number and identity of bats using a hibernaculum cannot always be directly observed; in these cases, other forms of evidence of occupancy can be used (e.g., acoustic data, genetic material, swarming behaviour). For example, at sites where there was appropriate habitat for hibernation but a hibernaculum could not be verified (e.g., chambers are inaccessible, or entry is avoided to minimize disturbance), swarming (an activity that typically occurs adjacent to or within a hibernaculum in the fall) was considered an indicator of a site’s use for hibernation. In addition, bat activity in the spring during the period when bats emerge from hibernacula may also be used as an indicator of the sites’ occupancy. As such, these sites (i.e., the subterranean features described in the biophysical attributes) were included in the identification of critical habitat. A threshold number of individuals is not defined in the occupancy criterion due to the difficulty in locating individuals within hibernacula and obtaining accurate counts, while also recognizing that the discovery of one hibernating individual often indicates the occurrence of more undetected individuals using the hibernaculum.
Due to the difficulty in distinguishing Little Brown Myotis from Yuma Myotis within areas where the ranges of these two species overlap and the extreme risk that WNS presents to bat populations, records that were unable to differentiate the two were included in the identification of critical habitat as a precautionary measure. Similarly, it is often not possible to identify to acoustic recordings to the species’ level due to similar acoustic signatures among the species. However, acoustic data that could be identified to the Myotis level were used as supporting evidence for a sites’ inclusion as critical habitat (in conjunction with other factors such as time of year the recording was obtained, species’ present within the study area, etc.).
The time period (1995 - 2018) recognizes that Little Brown Myotis, Northern Myotis, and Tri-colored Bat exhibit strong site fidelity to hibernacula, individual sites may be used by hibernating bats for decades, and suitable hibernacula may be limiting.
Sites that were deemed to have unsuitable habitat (e.g., suitable habitat no longer exists, or hibernating bats did not survive winter) were not identified as critical habitat; this does not include sites affected by WNS (occupied or previously-occupied). The long-term persistence of the WNS pathogen, P. destructans, in hibernacula affects the suitability of caves and mines for maintaining self-sustaining and resilient bat populations. Nevertheless, WNS-affected hibernacula are considered critical habitat because they need to be preserved from loss or modification to aid population recovery in the scenario that a treatment or decontamination measures are discovered, or natural resistance is developed.
Knowledge regarding the importance of habitat associations, their configuration surrounding the hibernacula, and at what geographical scale they exert an influence, if any, on the predictability of bat occurrence at these sites, is limited. At present, knowledge related to the locations of hibernacula is also limited throughout the range of these species. In addition, many potentially suitable overwintering locations require evidence of use by hibernating bats. Filling these knowledge gaps is necessary to complete the identification of critical habitat for hibernacula and will be addressed through completion of the schedule of studies (section 7.2).
Biophysical attributes
- Hibernacula for these species are subterranean features, such as caves, abandoned mines, hand-dug wells, cellars, tunnels, rock crevices or tree root hollows where light and noise levels are low
- Hibernacula typically contain sections that have relatively stable temperatures (2-10 °C) and stable, high humidity levels (>80 %)
A single hibernaculum may include multiple entry and exit points and vast underground networks of chambers. The full extent of these features are included in the definition of hibernacula as critical habitat (regardless of the location of hibernating bats within the structure) because (1) individuals may use multiple areas within these structures and it is not always possible to determine their usage, and (2) the entire, intact network is generally required to maintain microclimatic conditions (e.g., air flow, temperature, and humidity).
Sites used for hibernation in western and northern Canada, particularly along the Pacific Coast, may differ substantially from those elsewhere in the species Canadian range, and our knowledge of the location and biophysical attributes of these hibernacula is very limited (Jung et al. 2014). Bats in some parts of British Columbia may employ different strategies to survive the winter; information on winter activity patterns and how bats use overwintering sites in these areas is limited. However, based on the best available information, the occupancy criteria and biophysical attributes described above are used to identify critical habitat for western Canada herein until further research determines additional or alternative considerations. The schedule of studies (section 7.2) will allow for the complete identification of critical habitat for hibernacula in these areas.
Geographical location
The areas known to contain critical habitat for Little Brown Myotis, Northern Myotis, and/or Tri-colored Bat are presented in Figures 4-13. Critical habitat occurs within the standardized UTM grids where the critical habitat criteria (occupancy and biophysical attributes) described in this section are met. Critical habitat is displayed as UTM grids that represent all species that occur within a particular province or territory and identified critical habitat may include one or more of the species of interest (e.g., areas outside of the Tri-colored Bat documented range represents critical habitat for Little Brown Myotis and/or Northern Myotis). The UTM grid squares shown on these figures are part of a standardized national grid system that highlights the general geographic area containing critical habitat. Critical habitat is presented within standardized UTM grids, in order to respect protocols for provincial and territorial species at risk data use (and related agreements) and to protect the species and their habitats from disturbance and the potential introduction of WNS. The grid size used to display the critical habitat (i.e., 10, 50, or 100 km2) is relative to the risks associated with the location of critical habitat being discovered (as perceived by the data owners). More detailed information on the location of critical habitat to support protection of the species and its habitat may be requested, on a need-to-know basis, by contacting Environment and Climate Change Canada’s Recovery Planning section at: ec.planificationduretablissement-recoveryplanning.ec@canada.ca. An agreement with the province/territory or other data custodian may be required to share information on the detailed locations of hibernacula outside of Environment and Climate Change Canada.
A total of 233 hibernacula were identified as critical habitat in Newfoundland and Labrador, Nova Scotia, New Brunswick, Quebec, Ontario, Manitoba, Alberta, British Columbia, and Northwest Territories based on data as of May 2018. No hibernacula are known in Yukon, Saskatchewan, and Nunavut. Data on the locations of hibernacula in Prince Edward Island are unavailable because of landowner privacy concerns. Additional critical habitat may be added in the future as new information becomes available in an amended recovery strategy or in the species’ action plan.
Maternity roosts
Maternity roosts are used for giving birth and rearing young, and clearly contribute to the survival and recovery of these three species of bats. However, the locations of the vast majority of maternity roosts are currently either unknown or undocumented, or the data are unavailable to Environment and Climate Change Canada. Given this, it is not possible to determine which maternity roosts are necessary for the survival or recovery of these species; therefore, maternity roosts are not identified as critical habitat in this recovery strategy.
Once additional information on the locations and attributes of maternity roosts is available, it will be possible to set criteria that would identify the roosts that are necessary for the survival or recovery of the species as critical habitat. Criteria for identifying which maternity roosts are critical habitat would likely consider species, number of individuals using the roost, whether the roost is within a WNS-affected area, and the number of other known maternity roosts in the vicinity.
The work required to obtain the necessary information and establish criteria for the identification of maternity roosts as critical habitat is included in the schedule of studies (section 7.2).
Similar to hibernacula, knowledge of the location and biophysical attributes of maternity roosts in western Canada is very limited and habitat characteristics may differ substantially from those elsewhere in the species’ Canadian range (Jung et al. 2014). Filling these knowledge gaps is necessary to complete the identification of critical habitat for maternity roosts and will be addressed through completion of the schedule of studies (section 7.2).
Landscape-scale identification
The geographic range of the species, threats, and habitat specificity of Little Brown Myotis (and possibly Northern Myotis) suggest the critical habitat associated with summering habitat (i.e., roosting and foraging) should eventually be identified at a landscape scale. This would permit the long-term management of the habitat needed for survival and recovery of the species. This type of identification would reflect the dynamic mosaic of habitat conditions available and required on the landscape. Nevertheless, the available information is not adequate to currently identify critical habitat at a landscape scale for the following reasons:
- There is a lack of data related to bat presence and abundance in large portions of their ranges
- Habitat requirements may vary across the ranges of the species. Management units (i.e., geographic units within which critical habitat would be managed) need to be identified in such a way to best reflect variation in habitat use and management patterns
- There is a lack of understanding and data to indicate the appropriate configuration of important landscape biophysical attributes
- It is unclear whether certain summering habitats with specific biophysical attributes may be functionally more important than others. For example, specific habitats may have greater densities of individuals and/or result in higher reproductive success
- The relationships between anthropogenic disturbance and habitat quality are poorly known. A better understanding of these relationships is needed to ensure sufficient suitable habitat is available for these species and to identify at what scale and intensity activities would be likely to destroy the critical habitat
It will take many years to gather sufficient data to address the knowledge gaps above. Therefore, a long-term schedule of studies is presented in section 7.2 to address the landscape-scale identification of summering habitat.
Other habitats
Currently, male roosting sites, migration routes, and swarming sites are not identified as critical habitat. It is unclear whether the habitats that support male roosts or migration routes would be required for the survival or recovery of each species. At present, the knowledge of habitat requirements for the selection of significant swarming sites is insufficient to identify the biophysical attributes. Determining if male roosting sites and migration routes warrant identification as critical habitat and studies on the importance and biophysical attributes of swarming sites is included in the schedule of studies (section 7.2).
In summary, the critical habitat of Little Brown Myotis, Northern Myotis, and Tri-colored Bat can be partially identified at this time. A schedule of studies has been developed to provide the information necessary to complete the identification of critical habitat that will be sufficient to meet the population and distribution objectives. Although the short-term schedule of studies spans six years, the critical habitat can be updated as new information becomes available, either in a revised recovery strategy or action plan(s).

Long description
Standardized universal transverse mercator (UTM) grid square (red border and semi-transparent fill) at a 50 x 50 km resolution containing critical habitat for Little Brown Myotis (Myotis lucifugus) and/or Northern Myotis (Myotis septentrionalis) in Newfoundland and Labrador. The grid square covers both the eastern and western portions of White Bay on the Island of Newfoundland. The standardized national grid system indicates the geographic area containing critical habitat but detailed mapping is not shown. Critical habitat for these species occurs within this UTM grid square where the description of critical habitat is met (i.e., hibernacula have been identified).

Long description
Grid squares (red border and semi-transparent fill) containing critical habitat for Little Brown Myotis (Myotis lucifugus), Northern Myotis (Myotis septentrionalis), and/or Tri-colored Bat (Perimyotis subflavus) in Nova Scotia. Critical habitat for these species occurs within the 10 x 10 km standardized UTM grid squares where the description of critical habitat is met (i.e., hibernacula have been identified). Standardized UTM grid squares at the intersection of UTM zones are merged with their adjacent grid squares. This standardized national grid system indicates the general geographic area containing critical habitat; detailed critical habitat mapping is not shown. Six groups (labelled 5A to 5F) contain a total of 19 grids containing cirtical habitat for these species. One group is found in the southeastern part of Cape Breton Island and five groups are located in central Nova Scotia.

Long description
Grid squares (five) (red border and semi-transparent fill) containing critical habitat for Little Brown Myotis (Myotis lucifugus), Northern Myotis (Myotis septentrionalis), and/or Tri-colored Bat (Perimyotis subflavus) in northern Nova Scotia. Critical habitat for these species occurs within these 10 x 10 km standardized UTM grid squares where the description of critical habitat is met (i.e., hibernacula have been identified). This standardized national grid system indicates the general geographic area containing critical habitat; detailed critical habitat mapping is not shown. Grid squares containing critical habitat are located in the northeastern portion of Nova Scotia, south of Cape Breton.

Long description
Grid square (red border and semi-transparent fill) containing critical habitat for Little Brown Myotis (Myotis lucifugus), Northern Myotis (Myotis septentrionalis), and/or Tri-colored Bat (Perimyotis subflavus) in mainland Nova Scotia. Critical habitat for these species occurs within the 10 x 10 km standardized UTM grid square where the description of critical habitat is met (i.e., hibernacula have been identified). This standardized national grid system indicates the general geographic area containing critical habitat; detailed critical habitat mapping is not shown. The grid square is located east of Truro.

Long description
Grid squares (three) (red border and semi-transparent fill) that contain critical habitat for Little Brown Myotis (Myotis lucifugus), Northern Myotis (Myotis septentrionalis), and/or Tri-colored Bat (Perimyotis subflavus) in mainland Nova Scotia. Critical habitat for these species occurs within these 10 x 10 km standardized UTM grid squares where the description of critical habitat is met (i.e., hibernacula have been identified). This standardized national grid system indicates the general geographic area containing critical habitat; detailed critical habitat mapping is not shown. Grid squares containing critical habitat are located in the central portion of Nova Scotia, south of Truro.

Long description
Grid squares (three) (red border and semi-transparent fill) containing critical habitat for Little Brown Myotis (Myotis lucifugus), Northern Myotis (Myotis septentrionalis), and/or Tri-colored Bat (Perimyotis subflavus) in mainland Nova Scotia . Critical habitat for these species occurs within these 10 x 10 km standardized UTM grid squares where the description of critical habitat is met (i.e., hibernacula have been identified). This standardized national grid system indicates the general geographic area containing critical habitat; detailed critical habitat mapping is not shown. Grid squares containing critical habitat are located in the central portion of Nova Scotia, west of Truro and north of Halifax.

Long description
Grid squares (five) (red border and semi-transparent fill) containing critical habitat for Little Brown Myotis (Myotis lucifugus), Northern Myotis (Myotis septentrionalis), and/or Tri-colored Bat (Perimyotis subflavus) in mainland Nova Scotia . Critical habitat for these species occurs within these 10 x 10 km standardized UTM grid squares where the description of critical habitat is met (i.e., hibernacula have been identified). This standardized national grid system indicates the general geographic area containing critical habitat; detailed critical habitat mapping is not shown. Grid squares containing critical habitat are located in the central portion of Nova Scotia, northwest of Halifax.

Long description
Grid squares (two) (red border and semi-transparent fill) containing critical habitat for Little Brown Myotis (Myotis lucifugus), Northern Myotis (Myotis septentrionalis), and/or Tri-colored Bat (Perimyotis subflavus) in Nova Scotia. Critical habitat for these species occurs within these 10 x 10 km standardized UTM grid squares where the description of critical habitat is met (i.e., hibernacula have been identified). This standardized national grid system indicates the general geographic area containing critical habitat; detailed critical habitat mapping is not shown. Grid squares containing critical habitat are located in the western portion of Nova Scotia, northeast of Digby.

Long description
Grid square (red border and semi-transparent fill) containing critical habitat for Little Brown Myotis (Myotis lucifugus), Northern Myotis (Myotis septentrionalis), and/or Tri-colored Bat (Perimyotis subflavus) on Prince Edward Island. Critical habitat for these species occurs within these 10 x 10 km standardized UTM grid squares where the description of critical habitat is met (i.e., hibernacula have been identified). This standardized national grid system indicates the general geographic area containing critical habitat; detailed critical habitat mapping is not shown. The grid square containing critical habitat is located in the central portion of the northern coast of Prince Edward Island.

Long description
Grid squares (nine) containing critical habitat for Little Brown Myotis (Myotis lucifugus), Northern Myotis (Myotis septentrionalis), and/or Tri-colored Bat (Perimyotis subflavus) in southern New Brunswick. Critical habitat for these species occurs within these 10 x 10 km standardized UTM grid squares where the description of critical habitat is met (i.e., hibernacula have been identified). This standardized national grid system indicates the general geographic area containing critical habitat; detailed critical habitat mapping is not shown. Three groups of grid squares (indicated by letters A to C) are located between Shepody Bay and Saint John.

Long description
Grid squares (four) containing critical habitat for Little Brown Myotis (Myotis lucifugus), Northern Myotis (Myotis septentrionalis), and/or Tri-colored Bat (Perimyotis subflavus) in southern New Brunswick (Shepody Bay area). Critical habitat for these species occurs within these 10 x 10 km standardized UTM grid squares where the description of critical habitat is met (i.e., hibernacula have been identified). This standardized national grid system indicates the general geographic area containing critical habitat; detailed critical habitat mapping is not shown. Grid squares (three) containing critical habitat extend continuously from the mouth of Shepody Bay and from Peticodiac River east to Dorchester.

Long description
Grid squares (four) containing critical habitat for Little Brown Myotis (Myotis lucifugus), Northern Myotis (Myotis septentrionalis), and/or Tri-colored Bat (Perimyotis subflavus) in southeastern New Brunswick (Sussex area). Critical habitat for these species occurs within these 10 x 10 km standardized UTM grid squares where the description of critical habitat is met (i.e., hibernacula have been identified). This standardized national grid system indicates the general geographic area containing critical habitat; detailed critical habitat mapping is not shown. Critical habitat grid squares encompass an area south of Jeffries Corner along the Hammond River and an area east of Sussex Corner along Trout Creek.

Long description
Grid square (red border and semi-transparent fill) containing critical habitat for Little Brown Myotis (Myotis lucifugus), Northern Myotis (Myotis septentrionalis), and/or Tri-colored Bat (Perimyotis subflavus) in south-central New Brunswick, encompassing the greater Saint region, and the southern portion of the Kingston Peninsula. Critical habitat for these species occurs within this 10 x 10 km standardized UTM grid square where the description of critical habitat is met (i.e., hibernacula have been identified). This standardized national grid system indicates the general geographic area containing critical habitat; detailed critical habitat mapping is not shown. Standardized UTM grid squares at the intersection of UTM zones are merged with their adjacent grid squares.

Long description
Grid squares (20) containing critical habitat for Northern Myotis (Myotis septentrionalis), Little Brown Myotis (Myotis luifugus), and/or Tri-colored Bat (Perimyotis subflavus) in Quebec. The locations of the critical habitat grid squares are isolated and are widely dispersed from one another. Critical habitat for these species occurs within these 50 x 50 km standardized UTM grid squares where the description of critical habitat is met (i.e., hibernacula have been identified). This standardized national grid system indicates the general geographic area containing critical habitat; detailed critical habitat mapping is not shown. Six groups of UTM grid squares are shown (labelled 8A to 8F).

Long description
Grid squares (three) containing critical habitat for Northern Myotis (Myotis septentrionalis), Little Brown Myotis (Myotis luifugus), and/or Tri-colored Bat (Perimyotis subflavus) in Quebec, along the southe shoreof the mouth of the St. Lawrence River (Bas-Saint-Laurent regionarea). The locations of the critical habitat grid squares are isolated and are widely dispersedfrom one another. Critical habitat for these species occurs within these 50 x 50 km standardized UTM grid squares where the description of critical habitat is met (i.e., hibernacula have been identified). This standardized national grid system indicates the general geographic area containing critical habitat; detailed critical habitat mapping is not shown.

Long description
Grid squares (six) containing critical habitat for Little Brown Myotis (Myotis lucifugus), Northern Myotis (Myotis septentrionalis), and/or Tri-colored Bat (Perimyotis subflavus) in Quebec, in the Eastern Townships region. Critical habitat grid squares are widely dispersed in the region, with three grids representing adjacent geographic areas where critical habitat has been identified. Critical habitat for these species occurs within these 50 x 50 km standardized UTM grid squares where the description of critical habitat is met (i.e., hibernacula have been identified). This standardized national grid system indicates the general geographic area containing critical habitat; detailed critical habitat mapping is not shown.

Long description
Grid squares (two) containing critical habitat for Little Brown Myotis (Myotis lucifugus), Northern Myotis (Myotis septentrionalis), and/or Tri-colored Bat (Perimyotis subflavus) in Quebec, in the Lac Saint-Jean and Laurentian Mountains regions. Critical habitat grid squares are widely dispersed in the region, with three grids representing adjacent geographic areas where critical habitat has been identified. Critical habitat for these species occurs within these 50 x 50 km standardized UTM grid squares where the description of critical habitat is met (i.e., hibernacula have been identified). This standardized national grid system indicates the general geographic area containing critical habitat; detailed critical habitat mapping is not shown.

Long description
Grid squares (six) containing critical habitat for Little Brown Myotis (Myotis lucifugus), Northern Myotis (Myotis septentrionalis), and/or Tri-colored Bat (Perimyotis subflavus) in southern Quebec. Critical habitat grid squares are widely dispersed in the region, with three grids representing adjacent geographic areas where critical habitat has been identified. Critical habitat for these species occurs within these 50 x 50 km standardized UTM grid squares where the description of critical habitat is met (i.e., hibernacula have been identified). This standardized national grid system indicates the general geographic area containing critical habitat; detailed critical habitat mapping is not shown. The grid squares cover the area from Maniwaki south to Ottawa and surrounding regions.

Long description
Grid square containing critical habitat for Little Brown Myotis (Myotis lucifugus), Northern Myotis (Myotis septentrionalis), and/or Tri-colored Bat (Perimyotis subflavus) in Quebec. Critical habitat grid squares are widely dispersed in the region, with three grids representing adjacent geographic areas where critical habitat has been identified. Critical habitat for these species occurs within these 50 x 50 km standardized UTM grid squares where the description of critical habitat is met (i.e., hibernacula have been identified). This standardized national grid system indicates the general geographic area containing critical habitat; detailed critical habitat mapping is not shown. The area covers a large portion of Lac Chibougamau, directly west of Alma.

Long description
Grid squares (two) containing critical habitat for Northern Myotis (Myotis septentrionalis), Little Brown Myotis (Myotis lucifugus), and/or Tri-colored Bat (Perimyotis subflavus) in Quebec. Critical habitat grid squares are located near Val-d’Or and north of Lebel-sur-Quevillon. Critical habitat for these species occurs within these 50 x 50 km standardized UTM grid squares where the description of critical habitat is met (i.e., hibernacula have been identified). This standardized national grid system indicates the general geographic area containing critical habitat; detailed critical habitat mapping is not shown.

Long description
Grid squares (47) (red border and semi-transparent fill) containing critical habitat for Little Brown Myotis (Myotis lucifugus), Northern Myotis (Myotis septentrionalis), and/or Tri-colored Bat (Perimyotis subflavus) in Ontario. Critical habitat grid squares are widely dispersed in the southern boreal forest of Ontario, with a high concentration in northeastern Ontario along the Quebec border, on the north shore of Lake Huron and in the central Ottawa Valley, around Algonquin Park; a single grid is located in southern Ontario between Lake Erie and Lake Ontario. Critical habitat for these species occurs within these 50 x 50 km standardized UTM grid squares where the description of critical habitat is met (i.e., hibernacula have been identified). This standardized national grid system indicates the general geographic area containing critical habitat; detailed critical habitat mapping is not shown. Standardized UTM grid squares at the intersection of UTM zones are merged with their adjacent grid squares. Fourteen groups of UTM grid squares (labelled 9A to 9N) are shown.

Long description
Grid squares (two) (red border and semi-transparent fill) containing critical habitat for Little Brown Myotis (Myotis lucifugus), Northern Myotis (Myotis septentrionalis), and/or Tri-colored Bat (Perimyotis subflavus) in Ontario, west of Ottawa and north of Kingston. Critical habitat for these species occurs within these 50 x 50 km standardized UTM grid squares where the description of critical habitat is met (i.e., hibernacula have been identified). This standardized national grid system indicates the general geographic area containing critical habitat; detailed critical habitat mapping is not shown. Standardized UTM grid squares at the intersection of UTM zones are merged with their adjacent grid squares. The grids cover the area from Arnprior west to Renfrew and south to Westport.

Long description
Grid squares (five) (red border and semi-transparent fill) containing critical habitat for Little Brown Myotis (Myotis lucifugus), Northern Myotis (Myotis septentrionalis), and/or Tri-colored Bat (Perimyotis subflavus) in Ontario, west of Kingston. Critical habitat for these species occurs within these 50 x 50 km standardized UTM grid squares where the description of critical habitat is met (i.e., hibernacula have been identified). This standardized national grid system indicates the general geographic area containing critical habitat; detailed critical habitat mapping is not shown. Standardized UTM grid squares at the intersection of UTM zones are merged with their adjacent grid squares. The grids cover the area from Peterborough north to Barry’s Bay and east to Verona.

Long description
Grid square (red border and semi-transparent fill) containing critical habitat for Little Brown Myotis (Myotis lucifugus), Northern Myotis (Myotis septentrionalis), and/or Tri-colored Bat (Perimyotis subflavus) in Ontario, in the Toronto-Hamilton region. Critical habitat for these species occurs within these 50 x 50 km standardized UTM grid squares where the description of critical habitat is met (i.e., hibernacula have been identified). This standardized national grid system indicates the general geographic area containing critical habitat; detailed critical habitat mapping is not shown. Standardized UTM grid squares at the intersection of UTM zones are merged with their adjacent grid squares. The area takes in Cambridge, Burlington, Brampton, and Guelph.

Long description
Grid squares (two) (red border and semi-transparent fill) containing critical habitat for Little Brown Myotis (Myotis lucifugus), Northern Myotis (Myotis septentrionalis), and/or Tri-colored Bat (Perimyotis subflavus) in Ontario, in the Nottawasaga Bay and Georgian Bay regions. Critical habitat for these species occurs within these 50 x 50 km standardized UTM grid squares where the description of critical habitat is met (i.e., hibernacula have been identified). This standardized national grid system indicates the general geographic area containing critical habitat; detailed critical habitat mapping is not shown. Standardized UTM grid squares at the intersection of UTM zones are merged with their adjacent grid squares.

Long description
Grid squares (four) (red border and semi-transparent fill) containing critical habitat for Little Brown Myotis (Myotis lucifugus), Northern Myotis (Myotis septentrionalis), and/or Tri-colored Bat (Perimyotis subflavus) in Ontario, in the North Bay region. Critical habitat for these species occurs within these 50 x 50 km standardized UTM grid squares where the description of critical habitat is met (i.e., hibernacula have been identified). This standardized national grid system indicates the general geographic area containing critical habitat; detailed critical habitat mapping is not shown. Standardized UTM grid squares at the intersection of UTM zones are merged with their adjacent grid squares.

Long description
Grid squares (three) (red border and semi-transparent fill) containing critical habitat for Little Brown Myotis (Myotis lucifugus), Northern Myotis (Myotis septentrionalis), and/or Tri-colored Bat (Perimyotis subflavus) in Ontario, in the Sudbury region. Critical habitat for these species occurs within these 50 x 50 km standardized UTM grid squares where the description of critical habitat is met (i.e., hibernacula have been identified). This standardized national grid system indicates the general geographic area containing critical habitat; detailed critical habitat mapping is not shown. Standardized UTM grid squares at the intersection of UTM zones are merged with their adjacent grid squares.

Long description
Grid squares (seven) (red border and semi-transparent fill) containing critical habitat for Little Brown Myotis (Myotis lucifugus), Northern Myotis (Myotis septentrionalis), and/or Tri-colored Bat (Perimyotis subflavus) in Ontario, in the Timmins region. Critical habitat for these species occurs within these 50 x 50 km standardized UTM grid squares where the description of critical habitat is met (i.e., hibernacula have been identified). This standardized national grid system indicates the general geographic area containing critical habitat; detailed critical habitat mapping is not shown. Standardized UTM grid squares at the intersection of UTM zones are merged with their adjacent grid squares.

Long description
Grid squares (six) (red border and semi-transparent fill) containing critical habitat for Little Brown Myotis (Myotis lucifugus), Northern Myotis (Myotis septentrionalis), and/or Tri-colored Bat (Perimyotis subflavus) in Ontario, in the Sault Ste. Marie region. Critical habitat for these species occurs within these 50 x 50 km standardized UTM grid squares where the description of critical habitat is met (i.e., hibernacula have been identified). This standardized national grid system indicates the general geographic area containing critical habitat; detailed critical habitat mapping is not shown. Standardized UTM grid squares at the intersection of UTM zones are merged with their adjacent grid squares.

Long description
Grid squares (five) (red border and semi-transparent fill) containing critical habitat for Little Brown Myotis (Myotis lucifugus), Northern Myotis (Myotis septentrionalis), and/or Tri-colored Bat (Perimyotis subflavus) in Ontario. Critical habitat for these species occurs within these 50 x 50 km standardized UTM grid squares where the description of critical habitat is met (i.e., hibernacula have been identified). This standardized national grid system indicates the general geographic area containing critical habitat; detailed critical habitat mapping is not shown. Standardized UTM grid squares at the intersection of UTM zones are merged with their adjacent grid squares. These grid squares cover the area from Timmins west to Chapleau.

Long description
Grid squares (two) (red border and semi-transparent fill) that contain critical habitat for Little Brown Myotis (Myotis lucifugus), Northern Myotis (Myotis septentrionalis), and/or Tri-colored Bat (Perimyotis subflavus) in Ontario, in the Wawa region. Critical habitat for these species occurs within these 50 x 50 km standardized UTM grid squares where the description of critical habitat is met (i.e., hibernacula have been identified). This standardized national grid system indicates the general geographic area containing critical habitat; detailed critical habitat mapping is not shown. Standardized UTM grid squares at the intersection of UTM zones are merged with their adjacent grid squares.

Long description
Grid squares (four) (red border and semi-transparent fill) containing critical habitat for Little Brown Myotis (Myotis lucifugus), Northern Myotis (Myotis septentrionalis), and/or Tri-colored Bat (Perimyotis subflavus) in Ontario, in the Nipigon region. Critical habitat for these species occurs within these 50 x 50 km standardized UTM grid squares where the description of critical habitat is met (i.e., hibernacula have been identified). This standardized national grid system indicates the general geographic area containing critical habitat; detailed critical habitat mapping is not shown. Standardized UTM grid squares at the intersection of UTM zones are merged with their adjacent grid squares.

Long description
Grid squares (four) (red border and semi-transparent fill) containing critical habitat for Little Brown Myotis (Myotis lucifugus), Northern Myotis (Myotis septentrionalis), and/or Tri-colored Bat (Perimyotis subflavus) in Ontario, in the Thunder Bay region. Critical habitat for these species occurs within these 50 x 50 km standardized UTM grid squares where the description of critical habitat is met (i.e., hibernacula have been identified). This standardized national grid system indicates the general geographic area containing critical habitat; detailed critical habitat mapping is not shown. Standardized UTM grid squares at the intersection of UTM zones are merged with their adjacent grid squares.

Long description
Grid squares (three) (red border and semi-transparent fill) containing critical habitat for Little Brown Myotis (Myotis lucifugus), Northern Myotis (Myotis septentrionalis), and/or Tri-colored Bat (Perimyotis subflavus) in Ontario, west of Thunder Bay. Critical habitat for these species occurs within these 50 x 50 km standardized UTM grid squares where the description of critical habitat is met (i.e., hibernacula have been identified). This standardized national grid system indicates the general geographic area containing critical habitat; detailed critical habitat mapping is not shown. Standardized UTM grid squares at the intersection of UTM zones are merged with their adjacent grid squares.

Long description
Grid squares (three) (red border and semi-transparent fill) containing critical habitat for Little Brown Myotis (Myotis lucifugus) and/or Northern Myotis (Myotis septentrionalis) in Ontario, in the Kenora region. Critical habitat for these species occurs within these 50 x 50 km standardized UTM grid squares where the description of critical habitat is met (i.e., hibernacula have been identified). This standardized national grid system indicates the general geographic area containing critical habitat; detailed critical habitat mapping is not shown. Standardized UTM grid squares at the intersection of UTM zones are merged with their adjacent grid squares.

Long description
Grid squares (eleven) (red border and semi-transparent fill) containing critical habitat for Little Brown Myotis (Myotis lucifugus) and/or Northern Myotis (Myotis septentrionalis) in Manitoba. Critical habitat grid squares are highly concentrated in the area northwest of Lake Winnipeg and north of Grand Rapids; more isolated grids are located north of Lake St. Martin and west of Fisher Bay. Critical habitat for this species occurs within these 10 x 10 km standardized UTM grid squares where the description of critical habitat is met (i.e., hibernacula have been identified). This standardized national grid system indicates the general geographic area containing critical habitat; detailed critical habitat mapping is not shown. Four groups of UTM grid squares (labelled 10A to 10D) are shown.

Long description
Grid square (red border and semi-transparent fill) containing critical habitat for Little Brown Myotis (Myotis lucifugus) and/or Northern Myotis (Myotis septentrionalis) in Manitoba, northwest of Heda/Grindstone Provincial Park. Critical habitat for these species occurs within these 10 x 10 km standardized UTM grid squares where the description of critical habitat is met (i.e., hibernacula have been identified). This standardized national grid system indicates the general geographic area containing critical habitat; detailed critical habitat mapping is not shown. Standardized UTM grid squares at the intersection of UTM zones are merged with their adjacent grid squares.

Long description
Grid square (red border and semi-transparent fill) containing critical habitat for Little Brown Myotis (Myotis lucifugus) and/or Northern Myotis (Myotis septentrionalis) in Manitoba, east of Heda/Grindstone Provincial Park. Critical habitat for these species occurs within these 10 x 10 km standardized UTM grid squares where the description of critical habitat is met (i.e., hibernacula have been identified). This standardized national grid system indicates the general geographic area containing critical habitat; detailed critical habitat mapping is not shown. Standardized UTM grid squares at the intersection of UTM zones are merged with their adjacent grid squares.

Long description
Grid squares (two) (red border and semi-transparent fill) containing critical habitat for Little Brown Myotis (Myotis lucifugus) and/or Northern Myotis (Myotis septentrionalis) in Manitoba. Critical habitat for these species occurs within these 10 x 10 km standardized UTM grid squares where the description of critical habitat is met (i.e., hibernacula have been identified). This standardized national grid system indicates the general geographic area containing critical habitat; detailed critical habitat mapping is not shown. Standardized UTM grid squares at the intersection of UTM zones are merged with their adjacent grid squares. These grids cover Gypsumville.

Long description
Grid squares (seven) (red border and semi-transparent fill) containing critical habitat for Little Brown Myotis (Myotis lucifugus) and/or Northern Myotis (Myotis septentrionalis) in Manitoba, east of The Pas. Critical habitat for these species occurs within these 10 x 10 km standardized UTM grid squares where the description of critical habitat is met (i.e., hibernacula have been identified). This standardized national grid system indicates the general geographic area containing critical habitat; detailed critical habitat mapping is not shown. Standardized UTM grid squares at the intersection of UTM zones are merged with their adjacent grid squares.

Long description
Grid squares (six) containing critical habitat for Little Brown Myotis (Myotis lucifugus) and/or Northern Myotis (Myotis septentrionalis) in Alberta. Critical habitat for this species occurs within these 10 x 10 km standardized UTM grid squares where the description of critical habitat is met (i.e., hibernacula have been identified). This standardized national grid system indicates the general geographic area containing critical habitat; detailed critical habitat mapping is not shown. Four groups of UTM grid squares (labelled 11A to 11D) are shown; three near Jasper National Park, and one in Wood Buffalo National Park.

Long description
Grid square containing critical habitat for Little Brown Myotis (Myotis lucifugus) and/or Northern Myotis (Myotis septentrionalis) in Alberta (Wood Buffalo National Park area). Critical habitat is mapped as a single isolated grid in the northeastern portion of Wood Buffalo National Park. Critical habitat for this species occurs within this 10 x 10 km standardized UTM grid square where the description of critical habitat is met (i.e., hibernacula have been identified). This standardized national grid system indicates the general geographic area containing critical habitat; detailed critical habitat mapping is not shown.

Long description
Grid squares (two) containing critical habitat for Little Brown Myotis (Myotis lucifugus) and/or Northern Myotis (Myotis septentrionalis) in Alberta. Critical habitat for this species occurs within these 10 x 10 km standardized UTM grid squares where the description of critical habitat is met (i.e., hibernacula have been identified). This standardized national grid system indicates the general geographic area containing critical habitat; detailed critical habitat mapping is not shown. One grid square covers part of the Bighorn Range, while the other covers part of the Brazeau Range.

Long description
Grid square containing critical habitat for Little Brown Myotis (Myotis lucifugus) and/or Northern Myotis (Myotis septentrionalis) in Alberta (Banff National Park area). Critical habitat for this species occurs within this 10 x 10 km standardized UTM grid square where the description of critical habitat is met (i.e., hibernacula have been identified). This standardized national grid system indicates the general geographic area containing critical habitat; detailed critical habitat mapping is not shown.

Long description
Grid squares (two) containing critical habitat for Little Brown Myotis (Myotis lucifugus) and/or Northern Myotis (Myotis septentrionalis) in Alberta (Jasper National Park area). Critical habitat for this species occurs within these 10 x 10 km standardized UTM grid squares where the description of critical habitat is met (i.e., hibernacula have been identified). This standardized national grid system indicates the general geographic area containing critical habitat; detailed critical habitat mapping is not shown.

Long description
Grid squares (seven) (red border and semi-transparent fill) containing critical habitat for Little Brown Myotis (Myotis lucifugus) and/or Northern Myotis (Myotis septentrionalis) in southern British Columbia. Four groups of UTM grid squares (labelled 12A to 12D) are shown. Two of these groups are located along the coast, while the other two are located inland. Critical habitat for this species occurs within these 50 x 50 km standardized UTM grid squares where the description of critical habitat is met (i.e., hibernacula have been identified). This standardized national grid system indicates the general geographic area containing critical habitat; detailed critical habitat mapping is not shown.

Long description
Grid square (red border and semi-transparent fill) containing critical habitat for Little Brown Myotis (Myotis lucifugus) and/or Northern Myotis (Myotis septentrionalis) in southern British Columbia (Glacier National Park area). Critical habitat for this species occurs within this 50 x 50 km standardized UTM grid square where the description of critical habitat is met (i.e., hibernacula have been identified). This standardized national grid system indicates the general geographic area containing critical habitat; detailed critical habitat mapping is not shown.

Long description
Grid squares (two) (red border and semi-transparent fill) containing critical habitat for Little Brown Myotis (Myotis lucifugus) and/or Northern Myotis (Myotis septentrionalis) in southern British Columbia. Critical habitat for this species occurs within these 50 x 50 km standardized UTM grid squares where the description of critical habitat is met (i.e., hibernacula have been identified). This standardized national grid system indicates the general geographic area containing critical habitat; detailed critical habitat mapping is not shown. The area extends from Fort St. John west to Hudson’s Hope.

Long description
Grid squares (two) (red border and semi-transparent fill) containing critical habitat for Little Brown Myotis (Myotis lucifugus) in southern British Columbia. Critical habitat for this species occurs within this 50 x 50 km standardized UTM grid squares where the description of critical habitat is met (i.e., hibernacula have been identified). This standardized national grid system indicates the general geographic area containing critical habitat; detailed critical habitat mapping is not shown. The area is located on the coast of southern British Columbia. One grid covers Port Renfrew, and the other Port Alberni.

Long description
Grid squares (two) (red border and semi-transparent fill) containing critical habitat for Little Brown Myotis (Myotis lucifugus) in southern British Columbia. Critical habitat for this species occurs within this 50 x 50 km standardized UTM grid squares where the description of critical habitat is met (i.e., hibernacula have been identified). This standardized national grid system indicates the general geographic area containing critical habitat; detailed critical habitat mapping is not shown. The area is located on the coast of southern British Columbia and extends over a portion of the Vancouver Island ranges.

Long description
Grid squares (two) (red border and semi-transparent fill) containing critical habitat for Little Brown Myotis (Myotis lucifugus) in the southern Northwest Territories. Two groups of UTM grid squares (labelled 13A and 13B) are shown. The first group is located at the border between the Northwest Territories and Alberta. The second is located south of the border between the Northwest Territories and Yukon. Critical habitat for these species occurs within these 100 x 100 km standardized UTM grid squares where the description of critical habitat is met (i.e., hibernacula have been identified). This standardized national grid system indicates the general geographic area containing critical habitat; detailed critical habitat mapping is not shown.

Long description
Grid square (red border and semi-transparent fill) containing critical habitat for Little Brown Myotis (Myotis lucifugus) in the southern Northwest Territories (Wood Buffalo National Park area). Critical habitat for these species occurs within this 100 x 100 km standardized UTM grid square where the description of critical habitat is met (i.e., hibernacula have been identified). This standardized national grid system indicates the general geographic area containing critical habitat; detailed critical habitat mapping is not shown.

Long description
Grid square (red border and semi-transparent fill) containing critical habitat for Little Brown Myotis (Myotis lucifugus) in the southern Northwest Territories (Nahanni National Park Reserve area). Critical habitat for these species occurs within this 100 x 100 km standardized UTM grid square where the description of critical habitat is met (i.e., hibernacula have been identified). This standardized national grid system indicates the general geographic area containing critical habitat; detailed critical habitat mapping is not shown.
7.2 Schedule of studies to identify critical habitat
A schedule of studies has been developed to provide the information necessary to complete the identification of critical habitat (Table 6 & 7). A short-term schedule of studies (Table 6) has been developed to target those studies that can further the identification of critical habitat in the near-term to support achieving the short-term population and distribution objectives in Canada. A long-term schedule of studies (Table 7) has been developed to identify those studies that will support a landscape approach to the identification of additional critical habitat necessary for self-sustaining, resilient, redundant and representative bat populations in Canada.
Description of Activity | Rationale | Timeline |
---|---|---|
Work with researchers, provinces / territories, Aboriginal communities, and non-government organizations to determine how sensitive data can be made available and used for critical habitat identification. | Lack of data availability (particularly related to anthropogenic structures) is a major impediment to critical habitat identification. | 2019 |
Conduct surveys in areas where hibernacula and maternity roosts are suspected but unconfirmed. | Known locations of hibernacula and maternity roosts are limited. Many potentially suitable overwintering and maternity roost locations have evidence of use that is either dated or unconfirmed. Confirming use is needed to fully identify critical habitat. | 2019-2024 |
Conduct surveys to determine the extent of the species’ ranges where it is currently unknown (e.g., in the north). | The spatial extent of the species’ ranges, including locations of hibernacula and maternity roosts, is required to fully identify critical habitat. | 2019-2024 |
Refine biophysical attributes and criteria for hibernacula and determine biophysical attributes for maternity roosts (particularly in western and northern parts of the species’ ranges). | Although general biophysical attributes are understood, further refining these is deemed essential for full identification of critical habitat. Understanding overwintering behaviour and how bats use features to survive the winter is also required. Knowledge limitations are especially evident in western and northern parts of the species’ ranges. | 2019-2024 |
Develop and implement methods to identify individuals resistant to WNS. | If resistance to WNS is found in the population, evaluate how such findings would influence approaches to critical habitat identification. | 2019-2024 |
Identify the location, characteristics, and biophysical attributes of swarming sites. | The importance of individual swarming sites to survival and recovery and the important biophysical attributes of these sites are unknown. | 2019-2024 |
Determine criteria for identifying maternity roosts as critical habitat. | There is a lack of appropriate data to develop science-based criteria. | As appropriate |
Description of Activity | Rationale | Timeline |
---|---|---|
Increase surveys and monitoring of summering habitat at strategic locations. | Information on abundance and other measures of summering habitat quality is poor in many regions of the country. Increased surveys and monitoring in pre-determined locations is necessary. | 2019-2028 |
Determine male roosting sites and migration routes and whether they are considered necessary for recovery and survival, and thus would warrant critical habitat identification. | It is currently unclear if these habitats (or even a subset of these) should be considered critical habitat. | 2019-2024 |
Determine the appropriate configuration of landscape biophysical attributes, including investigation into relationships between habitat configuration and WNS transmission/prevalence. | To identify critical habitat at a landscape scale it is necessary to understand the biophysical attributes required by the species’ at this scale and to determine how these should be configured to meet the species’ needs. If certain habitat configurations are found to reduce WNS transmission (or improve survival of individuals following WNS infection), consider targeting these areas for critical habitat identification. | 2019-2028 |
Determine habitat quality across the species’ ranges, including investigation into relationships between habitat quality and WNS transmission/prevalence. | Information on abundance, productivity and other measures of habitat quality may lead to the identification of areas that contribute disproportionately to the survival or recovery of these species. If certain habitat conditions are found to reduce WNS transmission (or improve survival of individuals following WNS infection), consider targeting these areas for critical habitat identification. | 2019-2028 |
Determine the scale and intensity at which suitable habitat would likely be destroyed by anthropogenic activities. | A better understanding of the relationship between anthropogenic disturbance and habitat quality is needed to ensure sufficient suitable habitat is available for these species and to identify at what scale and intensity activities would be likely to destroy critical habitat. | 2019-2028 |
Determine how much suitable summering habitat is required to meet the population and distribution objectives. | It is uncertain whether summering habitat is limiting in Canada. An assessment of whether there is sufficient habitat in Canada to meet the population and distribution objectives is required. | 2028 |
Develop and validate summering habitat models to determine where biophysical attributes are present in required quantity, quality and configuration across the species’ ranges to meet population and distribution objectives. | Results from studies listed above, will allow models to be built to identify the location, quantity, and quality of habitat that should be identified as summering critical habitat. | 2027-2032 |
7.3 Activities likely to result in the destruction of critical habitat
This subsection of a recovery strategy identifies activities that are likely to cause the destruction of critical habitat and provides information on how these activities impact critical habitat. Destruction of critical habitat is determined on a case-by-case basis. Destruction would result if part of the critical habitat were degraded, either permanently or temporarily, such that it would not serve its function when needed by the species. Destruction may result from single or multiple activities at one point in time or from the cumulative effects of one or more activities over time. Activities described below include those likely to cause destruction of critical habitat for the species; however, destructive activities are not limited to those listed.
Hibernacula
Activities likely to result in the destruction of hibernacula identified as critical habitat include, but are not limited to, the following: activities resulting in the introduction of WNS into hibernacula previously free of WNS, activities that result in collapsed walls or ceilings or flooding, or activities that result in the hibernaculum being inaccessible or unavailable to bats or alters the hibernaculum’s temperature, humidity, airflow, or other microclimatic characteristics outside of the range acceptable to the bat species for which critical habitat is identified.
Description of activity | Description of effect |
---|---|
Anyone entering a hibernacula (e.g., researchers, cavers, tourists) that does not follow proper decontamination protocols (i.e., Canadian National White-nose Syndrome Decontamination Protocol - Canadian Wildlife Health Cooperative 2015b) | May result in permanent or temporary direct destruction of habitat by introducing WNS. The introduction of WNS to hibernacula via these activities can occur any time of the year. |
Modifications to accommodate visitors (e.g., observation platform, altering entrance) | May result in permanent or temporary direct destruction of habitat or indirect effects (e.g., changes to microclimatic conditions, limit bat access to site). These modifications can occur any time of the year and result in destruction. |
Erection of physical barriers (e.g., doors, gates, buildings) leading to reduced bat access and/or useq | May result in permanent or temporary direct destruction of habitat and/or indirect effects (e.g., restricting bat access to site, changes to airflow, temperature, or other microclimatic characteristics). The erection of barriers can occur any time of the year and result in destruction. |
Filling wells or sealing mine/cave entrances | May result in permanent direct destruction of habitat by eliminating the hibernaculum features or limiting access by bats to such features. These activities can occur any time of the year and result in destruction. |
Intentional vandalism | May result in permanent or temporary direct destruction of habitat and/or indirect effects (e.g., restricting bat access to site, removing/damaging barriers that were intended to limit human access, changes to airflow, temperature, or other microclimatic characteristics). This activity can occur any time of the year and result in destruction. |
Activities that cause excessive disturbance (e.g., light, noise, vibrations or visitation), such as mining exploration and development, quarrying, excavating, blasting, caving, forestry, research, and other practices. | May result in temporary destruction of habitat by reducing the functionality of the hibernacula to provide for the survival of bats during overwintering periods. These activities can cause bats to arouse from torpor which in turn could cause a cascade of arousals in nearby bats and/or could cause the abandonment of a site. Arousals from excessive disturbance causes increased fat consumption (and premature energy depletion), starvation, reduced energy reserves for reproduction, and ultimately, death. These activities may occur outside the hibernaculum and still cause destruction and repeated visits over several consecutive days can have the most severe impacts. These activities can cause destruction during the overwintering period from September to May. |
Activities that alter the microclimate characteristics (e.g., airflow or hydrology), limits access (e.g., collapse, flood) or directly removes (e.g., excavation) the hibernaculum, such as quarrying, mining exploration and development, agriculture, forestry, dam construction, and other practices. | May result in permanent or temporary direct destruction of habitat and/or indirect effects (e.g., ceiling or wall collapse, flooding, limit access to hibernaculum). These activities may occur outside the hibernaculum and at any time of the year and still cause destruction. |
q Bat-friendly gating is often necessary to prohibit human access to hibernacula. Any bat-friendly gates erected to restrict human access should be associated with a well-designed pre- and post-monitoring program that includes measures for adaptable management to ensure no negative impacts to the bats and ensure no reduction in bat access or use of the site (U.S. Fish and Wildlife Service 2007).
8 Measuring progress
The performance indicators presented below provide a way to define and measure progress toward achieving the population and distribution objectives.
Little Brown Myotis and Northern Myotis
- Little Brown Myotis’ and Northern Myotis’ extent of occurrence is maintained (or where applicable restored to) the pre-WNS extent (to be verified every five years)
- In the short-term (12-18 years) within areas affected by WNS, the declining population trends are stopped, or if feasible, increasing population trends are achieved. The objective will be measured against each 6-year cumulative interval (i.e., at 6 years [year 1 to 6 combined], at 12 years [year 1 to 12 combined] and at 18 years [year 1 to18 combined])
- In the long-term (many generations) within areas affected by WNS, each species’ population is self-sustaining, resilient, redundant and representative
- Within areas not yet known to be affected by WNS, stable population trends are maintained, or if feasible, increasing population trends are achieved
Tri-colored Bat
- Tri-colored Bat’s extent of occurrence is restored (then maintained) to the pre-WNS extent of occurrence (to be verified every five years)
- In the short-term (next 10 years), the declining population trend is stopped, or if feasible, an increasing population trend is achieved. The objective will be measured against each 5-year cumulative interval (i.e., at 5 years [year 1 to 5 combined] and at 10 years [year 1 to 10 combined])
- In the long-term (many generations), the population is self-sustaining, resilient, redundant and representative
9 Statement on action plans
One or more action plans for Little Brown Myotis, Northern Myotis, and Tri-colored Bat will be posted on the Species at Risk Public Registry within three years of the final posting of the recovery strategy.
10 References
Abbott, I. M., A. Berthinussen, E. Stone, M. Boonman, M. Melber, and J. Altringham. 2015. Bats and Roads. Pages 290-299 In R. van der Ree, D. J. Smith, and C. Grilo (eds.). Handbook of Road Ecology. John Wiley & Sons, Ltd. Chichester, UK.
Abbott, I. M., S. Harrison, and F. Butler. 2012. Clutter-adaptation of bat species predicts their use of under-motorway passageways of contrasting sizes – a natural experiment. Journal of Zoology 287(2): 124-132.
Adams, R. A. 2010. Bat reproduction declines when conditions mimic climate change projections for western North America. Ecology 91(8): 2437-2445.
Adams, R. A. and M. A. Hayes. 2008. Water availability and successful lactation by bats as related to climate change in arid regions of western North America. Journal of Animal Ecology 77(6): 1115-1121.
Amelon, S. and D. Burhans. 2006. Conservation assessment: Myotis septentrionalis (northern long-eared bat) in the Eastern United States. Pages 69-82 In F. Thompson (ed.). Conservation Assessements of Five Forest Bat Species in Eastern United States. United States Department of Agriculture Forest Service General Technical Report. NC.
Ancillotto, L., M. T. Serangeli, and D. Russo. 2013. Curiosity killed the bat: domestic cats as bat predators. Mammalian Biology 78(5): 369-373.
Anderson, D. M., P. M. Glibert, and J. M. Burkholder. 2002. Harmful algal blooms and eutrophication: nutrient sources, composition and consequences. Estuaries 25(4): 704-726.
Anderson, J. and C. Robert. 1971. A new unipolar electrode for electrocardiography in small mammals. Journal of Mammalogy 52: 469-471.
Anthony, E. L. and T. H. Kunz. 1977. Feeding strategies of the little brown bat, Myotis lucifugus, in southern New Hampshire. Ecology: 775-786.
Arlettaz, R., P. Christe, A. Lugon, N. Perrin, and P. Vogel. 2001. Food availability dictates the timing of parturition in insectivorous mouse‐eared bats. Oikos 95(1): 105-111.
Arnett, E., C. D. Hein, M. Schirmacher, M. Huso, and J. M. Szewczak. 2013a. Evaluating the effectiveness of an ultrasonic acoustic deterrent for reducing bat fatalities at wind turbines. PLoS One 8(9): e65794.
Arnett, E., G. Johnson, W. Erickson, and C. Hein. 2013b. A synthesis of operational mitigation studies to reduce bat fatalities at wind energy facilities in North America. National Renewable Energy Laboratory, Austin, TX.
Arnett, E. B., W. Brown, W. P. Erickson, J. K. Fiedler, B. L. Hamilton, T. H. Henry, A. Jain, G. D. Johnson, J. Kerns, and R. R. Koford. 2008. Patterns of bat fatalities at wind energy facilities in North America. The Journal of Wildlife Management 72(1): 61-78.
Arnett, E. B., M. Schirmacher, M. Huso, and J. Hayes. 2009. Effectiveness of changing wind turbine cut-in speed to reduce bat fatalities at wind facilities. Bats and Wind Energy Cooperative, Bat Conservation International, Austin, TX.
Baerwald, E. F., G. H. D'Amours, B. J. Klug, and R. M. Barclay. 2008. Barotrauma is a significant cause of bat fatalities at wind turbines. Current Biology 18(16): R695-R696.
Baerwald, E. F., J. Edworthy, M. Holder, and R. M. Barclay. 2009. A large‐scale mitigation experiment to reduce bat fatalities at wind energy facilities. The Journal of Wildlife Management 73(7): 1077-1081.
Ballmann, A. E., M. R. Torkelson, E. A. Bohuski, R. E. Russell, and D. S. Blehert. 2017. Dispersal Hazards of Pseudogymnoascus destructans by Bats and Human Activity at Hibernacula in Summer. J Wildl Dis.
Barbour, R. W. and W. H. Davis. 1969. Bats of America. University Press of Kentucky. Lexington, KY. 286 pp.
Barclay, R. and A. Kurta. 2007. Ecology and behaviour of bats roosting in tree cavities and under bark. Pages 17-60 In M. J. Lacki, J. P. Hayes, and A. Kurta (eds.). Bats in Forests: Conservation and Management. John Hopkins University Press. Baltimore, MD.
Barclay, R. R. and R. M. Brigham. 1996. Bats and Forests Symposium. British Columbia, Ministry of Forests Research Program. Victoria, BC.
BatsLive. 2015. Project EduBat. [accessed: 18 June 2015].
BC Ministry of Forests. 2003. Karst Management Handbook for British Columbia. Page 69 pp., Victoria, BC.
Bélanger, L. and M. Grenier. 2002. Agriculture intensification and forest fragmentation in the St. Lawrence valley, Quebec, Canada. Landscape Ecology 17(6): 495-507.
Bennett, V. J. and A. A. Zurcher. 2013. When corridors collide: road-related disturbance in commuting bats. The Journal of Wildlife Management 77(1): 93-101.
Boldogh, S., D. Dobrosi, and P. Samu. 2007. The effects of the illumination of buildings on house-dwelling bats and its conservation consequences. Acta Chiropterologica 9(2): 527-534.
Borkin, K., C. O’Donnell, and S. Parsons. 2011. Bat colony size reduction coincides with clear-fell harvest operations and high rates of roost loss in plantation forest. Biodiversity and Conservation 20(14): 3537-3548.
Both, C., C. A. Van Turnhout, R. G. Bijlsma, H. Siepel, A. J. Van Strien, and R. P. Foppen. 2009. Avian population consequences of climate change are most severe for long-distance migrants in seasonal habitats. Proceedings of the Royal Society B: Biological Sciences 277: 1259-1266.
Boyles, J. G. and D. P. Aubrey. 2006. Managing forests with prescribed fire: implications for a cavity-dwelling bat species. Forest Ecology and Management 222: 108-115.
Boyles, J. G. and V. Brack. 2009. Modeling survival rates of hibernating mammals with individual-based models of energy expenditure. Journal of Mammalogy 90(1): 9-16.
Briggler, J. T. and J. W. Prather. 2003. Seasonal use and selection of caves by the eastern pipistrelle bat (Pipistrellus subflavus). The American Midland Naturalist 149(2): 406-412.
Brigham, R. M. and M. B. Fenton. 1986. The influence of roost closure on the roosting and foraging behaviour of Eptesicus fuscus (Chiroptera: Vespertilionidae). Canadian Journal of Zoology 64(5): 1128-1133.
Broders, H. G., L. E. Burns, and S. C. McCarthy. 2013. First records of the Northern Myotis (Myotis septentrionalis) from labrador and summer distribution records and biology of little brown bats (Myotis lucifugus) in southern Labrador. Canadian Field-Naturalist 127(3): 266-269.
Broders, H. G., L. J. Farrow, R. N. Hearn, L. M. Lawrence, and G. J. Forbes. 2014. Stable isotopes reveal that little brown bats have a broader dietary niche than northern long-eared bats. Acta Chiropterologica 16(2): 315-325.
Broders, H. G. and G. J. Forbes. 2004. Interspecific and intersexual variation in roost-site selection of northern long-eared and little brown bats in the Greater Fundy National Park ecosystem. Journal of Wildlife Management 68(3): 602-610.
Broders, H. G., G. J. Forbes, S. Woodley, and I. D. Thompson. 2006. Range extent and stand selection for roosting and foraging in forest-dwelling Northern Long-Eared Bats and Little Brown Bats in the Greater Fundy Ecosystem, New Brunswick. The Journal of Wildlife Management 70(5): 1174-1184.
Broders, H. G., D. F. McAlpine, and G. J. Forbes. 2001. Status of the eastern pipistrelle (Pipistrellus subflavus) (Chiroptera: Vespertilionidae) in New Brunswick. Northeastern Naturalist 8(3): 331-336.
Broders, H. G., G. M. Quinn, and G. J. Forbes. 2003. Species status, and the spatial and temporal patterns of activity of bats in southwest Nova Scotia, Canada. Northeastern Naturalist 10(4): 383-398.
Brown, J. A., D. F. McAlpine, and R. Curley. 2007. Northern Long-eared Bat, Myotis septentrionalis (Chiroptera: Vespertilionidae), on Prince Edward Island: first records of occurrence and over-wintering. The Canadian Field-Naturalist 121(2): 208-209.
Buchalski, M. R., J. B. Fontaine, P. A. Heady III, J. P. Hayes, and W. F. Frick. 2013. Bat response to differing fire severity in mixed-conifer forest California, USA. PLoS One 8(3): e57884.
Bunkley, J. P., C. J. McClure, N. J. Kleist, C. D. Francis, and J. R. Barber. 2015. Anthropogenic noise alters bat activity levels and echolocation calls. Global Ecology and Conservation 3: 62-71.
Burles, D. W., M. B. Fenton, R. M. Barclay, R. M. Brigham, and D. Volkers. 2014. Aspects of the winter ecology of bats on Haida Gwaii, British Columbia. Northwestern Naturalist 95(3): 289-299.
Burns, L. E., T. R. Frasier, and H. G. Broders. 2014. Genetic connectivity among swarming sites in the wide ranging and recently declining little brown bat (Myotis lucifugus). Ecology and Evolution 4(21): 4130-4149.
Caceres, M. C. and R. M. Barclay. 2000. Myotis septentrionalis. Mammalian Species 634: 1-4.
Calvert, A. M., C. A. Bishop, R. D. Elliot, E. A. Krebs, T. M. Kydd, C. S. Machtans, and G. J. Robertson. 2013. A synthesis of human-related avian mortality in Canada. Avian Conservation and Ecology 8(2): 11.
Canadian Wildlife Federation. 2015. Help the Bats. Kanata, ON. [accessed: 19 June 2015].
Canadian Wildlife Federation. 2018. Little Brown Bat. [accessed: October 2018]
Canadian Wildlife Health Cooperative. 2015a. Bat White Nose Syndrome. [accessed: 22 April 2015].
Canadian Wildlife Health Cooperative. 2015b. Canadian National White-nose Syndrome Decontamination Protocol for Entering Bat Hibernacula. Canadian Wildlife Health Cooperative, Saskatoon, SkON.
Canadian Wildlife Health Cooperative. 2015c. A national plan to manage White Nose Syndrome in bats in Canada. Canadian Wildlife Health Cooperative: Canada's Inter-agency White Nose Syndrome Committee, Saskatoon, SkON.
Canadian Wind Energy Association. 2015. Canada's Current Installed Capacity.
Carr, J. A., R. F. Bernard, and W. H. Stiver. 2014. Unusual bat behavior during winter in Great Smoky Mountains National Park. Southeastern Naturalist 13(2): N18-N21.
Carter, T. C. and G. A. Feldhamer. 2005. Roost tree use by maternity colonies of Indiana bats and northern long-eared bats in southern Illinois. Forest Ecology and Management 219(2–3): 259-268.
Carter, T. C., W. M. Ford, and M. A. Menzel (eds.). 2002. Fire and bats in the Southeast and mid-Atlantic: more questions than answers? USDA Forest Service, Northeastern Research Station, Newton Square, PA.
Chaverri, G. and T. H. Kunz. 2011. Response of a specialist bat to the loss of a critical resource. PLoS One 6(12): e28821.
Chruszcz, B. and R. Barclay. 2002. Thermoregulatory ecology of a solitary bat, Myotis evotis, roosting in rock crevices. Functional Ecology 16(1): 18-26.
Clare, E. L., W. O. C. Symondson, H. Broders, F. Fabianek, E. E. Fraser, A. MacKenzie, A. Boughen, R. Hamilton, C. K. R. Willis, F. Martinez-Nuñez, A. K. Menzies, K. J. O. Norquay, M. Brigham, J. Poissant, J. Rintoul, R. M. R. Barclay, and J. P. Reimer. 2014. The diet of Myotis lucifugus across Canada: assessing foraging quality and diet variability. Molecular Ecology 23(15): 3618-3632.
Clark, D. R. and T. G. Lamont. 1976. Organochlorine residues in females and nursing young of the big brown bat (Eptesicus fuscus). Bulletin of Environmental Contamination and Toxicology 15(1): 1-8.
Coleman, J. L. and R. M. Barclay. 2011. Influence of urbanization on demography of little brown bats (Myotis lucifugus) in the prairies of North America. PLoS One 6(5): e20483.
Community Bat Programs of BC. 2014. Community Bat Programs Help People and Bats. [accessed: 22 April 2015].
Cornelison, C. T., M. K. Keel, K. T. Gabriel, C. K. Barlament, T. A. Tucker, G. E. Pierce, and S. A. Crow. 2014. A preliminary report on the contact-independent antagonism of Pseudogymnoascus destructans by Rhodococcus rhodochrous strain DAP96253. BMC Microbiology 14(1): 246.
COSEWIC. 2006. COSEWIC assessment and status report on the Rusty Blackbird Euphagus carolinus in Canada. Committee on the Status of Endangered Wildlife in Canada, Ottawa, ON.
COSEWIC. 2013. COSEWIC assessment and status report on the Little Brown Myotis Myotis lucifugus, Northern Myotis Myotis septentrionalis and Tri-colored Bat Perimyotis subflavus in Canada. Committee on the Status of Endangered Wildlife in Canada, Ottawa, ON.
Crampton, L. and R. Barclay. 1996. Habitat selection by bats in fragmented and unfragmented aspen mixedwood stands of different ages Page 292 In M. Brigham and R. Barclay, eds. Bats and Forests Symposium. BC Ministry of Forests Victoria, BC.
Cryan, P. M. 2011. Wind turbines as landscape impediments to the migratory connectivity of bats. Environmental Law 41: 355.
Cryan, P. M. and A. C. Brown. 2007. Migration of bats past a remote island offers clues toward the problem of bat fatalities at wind turbines. Biological Conservation 139(1): 1-11.
Cryan, P. M., C. U. Meteyer, J. G. Boyles, and D. S. Blehert. 2010. Wing pathology of white-nose syndrome in bats suggests life-threatening disruption of physiology. BMC Biology 8(1): 135.
CWHC. 2018. Canadian Wildlife Health Cooperative. CWHC, Saskatoon, SkON. [accessed: 29 May 2018].
Damm, J. P. and K. Geluso. 2008. Use of a mine by eastern pipistrelles (Perimyotis subflavus) in east central Nebraska. Western North American Naturalist 68(3): 382-389.
Darling, S. and R. Smith. 2011. Assessment of Vermont cave bat populations and proposal to list bat species. Vermont Fish and Wildlife Department, VT.
Davis, W. and H. Hitchcock. 1995. A new longevity record for the bat Myotis lucifugus. Bat Research News 36(1): 6.
Davis, W. H. 1970. Hibernation: ecology and physiological ecology. Biology of Bats 1: 265-300.
Davy, C. M., F. Martinez-Nuñez, C. K. R. Willis, and S. V. Good. 2015. Spatial genetic structure among bat hibernacula along the leading edge of a rapidly spreading pathogen. Conservation Genetics 16(5): 1013-1024.
de Groot, W. J., M. D. Flannigan, and A. S. Cantin. 2013. Climate change impacts on future boreal fire regimes. Forest Ecology and Management 294: 35-44.
Depew, D. C., N. M. Burgess, and L. M. Campbell. 2013. Modelling mercury concentrations in prey fish: derivation of a national-scale common indicator of dietary mercury exposure for piscivorous fish and wildlife. Environmental Pollution 176: 234-243.
Derusseau, S. N. and N. J. Huntly. 2012. Effects of gates on the nighttime use of mines by bats in northern Idaho. Northwestern Naturalist 93(1): 60-66.
Diamond, G. F. and J. M. Diamond. 2014. Bats and mines: evaluating Townsend's Big-Eared Bat (Corynorhinus townsendii) maternity colony behavioral response to gating. Western North American Naturalist 74(4): 416-426.
Dickenson, M. B., M. J. Lacki, and D. R. Cox. 2009. Fire and the endangered Indiana bat. Pages 51-75 In Fire in Eastern Oak Forests Conference. Northern Research Station, USDA. Newton Square, PA.
Dirzo, R., H. S. Young, M. Galetti, G. Ceballos, N. J. Isaac, and B. Collen. 2014. Defaunation in the Anthropocene. Science 345(6195): 401-406.
Dodd, L. E., M. J. Lacki, E. R. Britzke, D. A. Buehler, P. D. Keyser, J. L. Larkin, A. D. Rodewald, T. B. Wigley, P. B. Wood, and L. K. Rieske. 2012. Forest structure affects trophic linkages: how silvicultural disturbance impacts bats and their insect prey. Forest Ecology and Management 267: 262-270.
Douglas, M. R. and J. F. Tooker. 2015. Large-scale deployment of seed treatments has driven rapid increase in use of neonicotinoid insecticides and preemptive pest management in US field crops. Environmental Science & Technology 49(8): 5088-5097.
Dubois, J. E. and K. M. Monson. 2007. Recent distribution records of the Little Brown Bat, Myotis lucifugus, in Manitoba and Northwestern Ontario. The Canadian Field-Naturalist 121(1): 57-61.
Dzal, Y., L. A. Hooten, E. L. Clare, and M. Brock-Fenton. 2009. Bat activity and genetic diversity at Long Point, Ontario, an important bird stopover site. Acta Chiropterologica 11(2): 307-315.
Dzal, Y., L. P. McGuire, N. Veselka, and M. B. Fenton. 2011. Going, going, gone: the impact of white-nose syndrome on the summer activity of the little brown bat (Myotis lucifugus). Biology Letters 7(3): 392-394.
Edmonds, S. T., D. C. Evers, D. A. Cristol, C. Mettke-Hofmann, L. L. Powell, A. J. McGann, J. W. Armiger, O. P. Lane, D. F. Tessler, and P. Newell. 2010. Geographic and seasonal variation in mercury exposure of the declining Rusty Blackbird. The Condor 112(4): 789-799.
Eidels, R. R., J. O. Whitaker Jr, and D. W. Sparks. 2007. Insecticide residues in bats and guano from Indiana. Proceedings of the Indiana Academy of Science 116(1): 50-57.
Ellison, L., T. O’Shea, M. Bogan, A. Everette, and D. Schneider. 2003. Existing data on colonies of bats in the United States: summary and analysis of the US Geological Survey’s bat population database. Pages 127-237 In T. J. O'Shea and M. A. Bogan (eds.). Monitoring trends in bat populations of the United States and territories: problems and prospects. U.S. Geological Survey. Fort Collins, CO.
Entwistle, A. C., S. Harris, A. M. Hutson, P. Racey, A. Walsh, S. D. Gibson, I. Hepburn, and J. Johnston. 2001. Habitat management for bats: a guide for land managers, land owners and their advisors. Joint Nature Conservation Committee, Peterborough, UK.
Environment and Climate Change Canada. 2015. Pollution and Waste. Ottawa, ON. [accessed: June 2016].
Environment Canada. 2011. Presence and levels of priority pesticides in selected Canadian aquatic ecosystems. Water Science and Technology Directorate, Ottawa, ON.
Environment Canada. 2012. Recovery Strategy for the Woodland Caribou (Rangifer tarandus caribou), Boreal population, in Canada. Ottawa, ON.
Environment Canada. 2015. Recovery Strategy for Canada Warbler (Cardellina canadensis) in Canada [Proposed]. Ottawa, ON.
Environment Canada. 2016. Recovery Strategy for the Common Nighthawk (Chordeiles minor) in Canada. Ottawa, ON.
Ethier, K. and L. Fahrig. 2011. Positive effects of forest fragmentation, independent of forest amount, on bat abundance in eastern Ontario, Canada. Landscape Ecology 26(6): 865-876.
Fabianek, F., D. Gagnon, and M. Delorme. 2011. Bat distribution and activity in Montréal Island green spaces: responses to multi-scale habitat effects in a densely urbanized area. Ecoscience 18(1): 9-17.
Fabianek, F., M. A. Simard, E. B. Racine, and A. Desrochers. 2015. Selection of roosting habitat by male Myotis bats in a boreal forest. Canadian Journal of Zoology 93(7): 539.
Farrow, L. J. and H. G. Broders. 2011. Loss of forest cover impacts the distribution of the forest-dwelling tri-colored bat (Perimyotis subflavus). Mammalian Biology 76(2): 172-179.
Federal Provincial and Territorial Governments of Canada. 2010. Canadian Biodiversity: Ecosystem Status and Trends 2010. Ottawa, ON. [accessed: October 2015].
Fensome, A. G. and F. Mathews. 2016. Roads and bats: a meta-analysis and review of the evidence on vehicular collisions and barrier effects. Mammal Review Early View (online version of record published before inclusion in an issue).
Fenton, B. and R. M. R. Barclay. 1980a. Myotis lucifugus. Mammalian Species 142: 1-8.
Fenton, M. B. 1969. Summer activity of Myotis lucifugus (Chiroptera: Vespertilionidae) at hibernacula in Ontario and Quebec. Canadian Journal of Zoology 47(4): 597-602.
Fenton, M. B. 1970. A technique for monitoring bat activity with results obtained from different environments in southern Ontario. Canadian Journal of Zoology 48(4): 847-851.
Fenton, M. B. and R. M. Barclay. 1980b. Myotis lucifugus. Mammalian Species 142: 1-8.
Fitzgerald, W. F., D. R. Engstrom, R. P. Mason, and E. A. Nater. 1998. The case for atmospheric mercury contamination in remote areas. Environmental Science & Technology 32(1): 1-7.
Flannigan, M., B. Stocks, M. Turetsky, and M. Wotton. 2009. Impacts of climate change on fire activity and fire management in the circumboreal forest. Global Change Biology 15(3): 549-560.
Fleming, R., J.-N. Candau, and R. McAlpine. 2002. Landscape-scale analysis of interactions between insect defoliation and forest fire in central Canada. Climatic Change 55(1-2): 251-272.
Foster, R. and A. Kurta. 1999. Roosting ecology of the northern bat (Myotis septentrionalis) and comparisons with endangered Indiana bat (Myotis sodalis). Journal of Mammalogy 80: 659-672.
Francl, K. E., W. M. Ford, D. W. Sparks, and V. Brack Jr. 2012. Capture and reproductive trends in summer bat communities in West Virginia: assessing the impact of white-nose syndrome. Journal of Fish and Wildlife Management 3(1): 33-42.
Frank, C. L., A. Michalski, A. A. McDonough, M. Rahimian, R. J. Rudd, and C. Herzog. 2014. The resistance of a North American bat species (Eptesicus fuscus) to white-nose syndrome (WNS). PLoS One 9(12): e113958.
Frank, K. D. 1988. Impact of outdoor lighting on moths: an assessment. Journal of the Lepidopterists' Society 42(2): 63-93.
Fraser, E. E., L. P. McGuire, J. L. Eger, F. J. Longstaffe, and M. B. Fenton. 2012. Evidence of latitudinal migration in tri-colored bats, Perimyotis subflavus. PLoS One 7(2): e31419.
Freemark, K. E. and H. G. Merriam. 1986. Importance of area and habitat heterogeneity to bird assemblages in temperate forest fragments. Biological Conservation 36: 115-141.
Frick, W. F., J. F. Pollock, A. C. Hicks, K. E. Langwig, D. S. Reynolds, G. G. Turner, C. M. Butchkoski, and T. H. Kunz. 2010a. An emerging disease causes regional population collapse of a common North American bat species. Science 329(5992): 679-682.
Frick, W. F., D. S. Reynolds, and T. H. Kunz. 2010b. Influence of climate and reproductive timing on demography of little brown myotis Myotis lucifugus. Journal of Animal Ecology 79(1): 128-136.
Fujita, M. S. and T. H. Kunz. 1984. Pipistrellus subflavus. Mammalian Species 228: 1-6.
Fule, P. Z., A. E. Snyder, T. A. Heinlein, and W. W. Covington. 2004. Effects of an intense prescribed fire: is it ecological restoration? Restoration Ecology 12(2): 220-230.
Fuller, N. W., J. D. Reichard, M. L. Nabhan, S. R. Fellows, L. C. Pepin, and T. H. Kunz. 2011. Free-ranging little brown myotis (Myotis lucifugus) heal from wing damage associated with white-nose syndrome. EcoHealth 8(2): 154-162.
Furlonger, C., H. Dewar, and M. Fenton. 1987. Habitat use by foraging insectivorous bats. Canadian Journal of Zoology 65(2): 284-288.
Gaisler, J., V. Hanak, and I. Horacek. 1981. Remarks on the current status of bat populations in Czechoslovakia. Myotis 18(19): 68-75.
Garroway, C. J. and H. G. Broders. 2008. Day roost characteristics of northern long-eared bats (Myotis septentrionalis) in relation to female reproductive status. Ecoscience 15(1): 89-93.
Gaston, K. J., J. Bennie, T. W. Davies, and J. Hopkins. 2013. The ecological impacts of nighttime light pollution: a mechanistic appraisal. Biological Reviews 88(4): 912-927.
Gibbons, D., C. Morrissey, and P. Mineau. 2015. A review of the direct and indirect effects of neonicotinoids and fipronil on vertebrate wildlife. Environmental Science and Pollution Research 22(1): 103-118.
Girardin, M. P., A. A. Ali, C. Carcaillet, O. Blarquez, C. Hély, A. Terrier, A. Genries, and Y. Bergeron. 2013. Vegetation limits the impact of a warm climate on boreal wildfires. New Phytologist 199(4): 1001-1011.
GNWT. 2015a. NWT Species at Risk - Little Brown Myotis. Government of Northwest Territories, Yellowknife, NT. [accessed: 21 July 2015].
GNWT. 2015b. NWT Species at Risk - Northern Myotis. Government of the Northwest Territories, Yellowknife, NT. [accessed: 22 July 2015].
Goulson, D. 2013. Review: An overview of the environmental risks posed by neonicotinoid insecticides. Journal of Applied Ecology 50(4): 977-987.
Griffin, D. 1940. Migrations of New England bats. Bulletin of the Museum of Comparative Zoology 86: 217-246.
Grindal, S. D. 1996. Habitat use by bats in fragmented forests. Pages 260-272 In Bats and Forest Symposium. Research Branch, British Columbia Ministry of Forests. Victoria, BC.
Grindal, S. D. and R. M. Brigham. 1998. Short-term effects of small-scale habitat disturbance on activity by insectivorous bats. The Journal of Wildlife Management: 996-1003.
Grindal, S. D. and R. M. Brigham. 1999. Impacts of forest harvesting on habitat use by foraging insectivorous bats at different spatial scales. Ecoscience: 25-34.
Grindal, S. D., C. I. Stefan, and C. Godwin-Sheppard. 2011. Diversity, distribution, and relative abundance of bats in the oil sands regions of northeastern Alberta. Northwestern Naturalist 92(3): 211-220.
Grodsky, S. M., M. J. Behr, A. Gendler, D. Drake, B. D. Dieterle, R. J. Rudd, and N. L. Walrath. 2011. Investigating the causes of death for wind turbine-associated bat fatalities. Journal of Mammalogy 92(5): 917-925.
Haak, D. 2008. Sustainable Agriculture Land Management around Wetlands on the Canadian Prairies.In A. a. A.-F. Canada, ed. Agriculture and Agri-Food Canada, Ottawa, ON.
Hallegraeff, G. M. 1993. A review of harmful algal blooms and their apparent global increase. Phycologia 32(2): 79-99.
Harrison, J. B. 1965. Temperature effects on responses in the auditory system of the little brown bat Myotis l. lucifugus. Physiological Zoology: 34-48.
Harvey, M. J. 1992. Bats of the eastern United States. Arkansas Game and Fish Commission and United States Fish and Wildlife Service, Center for the Management, Utilization, and Protection of Water Resources, Tennessee Technological University, Cookeville, TN.
Harvey, M. J., J. S. Altenbach, and T. L. Best. 2011. Bats of the United States and Canada. Johns Hopkins University Press. Baltimore, MD. 202 pp.
Hayes, M. A. 2013. Bats killed in large numbers at United States wind energy facilities. BioScience 63(12): 975-979.
Hayhoe, K., C. P. Wake, T. G. Huntington, L. Luo, M. D. Schwartz, J. Sheffield, E. Wood, B. Anderson, J. Bradbury, and A. DeGaetano. 2007. Past and future changes in climate and hydrological indicators in the US Northeast. Climate Dynamics 28(4): 381-407.
Health Canada. 2013. Pest Control Product Sales Report for 2013.In H. Canada, ed., Ottawa, ON.
Hemmera. 2011. Raptor and Migratory Bird and Bat Monitoring and Follow-up Report 2010 and Recommendations for 2011. Hemmera, Vancouver, BC.
Henderson, L. E. and H. G. Broders. 2008. Movements and resource selection of the Northern Long-Eared Myotis (Myotis septentrionalis) in a forest-agriculture landscape. Journal of Mammalogy 89(4): 952-963.
Henderson, L. E., L. J. Farrow, and H. G. Broders. 2008. Intra-specific effects of forest loss on the distribution of the forest-dependent northern long-eared bat (Myotis septentrionalis). Biological Conservation 141(7): 1819-1828.
Henderson, L. E., L. J. Farrow, and H. G. Broders. 2009. Summer distribution and status of the bats of Prince Edward Island, Canada. Northeastern Naturalist 16(1): 131-140.
Henry, M., D. W. Thomas, R. Vaudry, and M. Carrier. 2002. Foraging distances and home range of pregnant and lactating Little Brown Bats (Myotis lucifugus). Journal of Mammalogy 83(3): 767-774.
Henshaw, R. E. and G. E. Folk. 1966. Relation of thermoregulation to seasonally changing microclimate in two species of bats (Myotis lucifugus and M. sodalis). Physiological Zoology: 223-236.
Hitchcock, H. B. 1949. Hibernation of bats in southeastern Ontario and adjacent Quebec. Canadian Field-Naturalist 63(1): 47-59.
Hitchcock, H. B. 1965. Twenty-three years of bat banding in Ontario and Quebec. Canadian Field-Naturalist 79: 4-14.
Hobson, K. A., E. M. Bayne, and S. L. Van Wilgenburg. 2002. Large-scale conversion of forest to agriculture in the boreal plains of Saskatchewan. Conservation Biology 16(6): 1530-1541.
Hogberg, L. K., K. J. Patriquin, and R. M. Barclay. 2002. Use by bats of patches of residual trees in logged areas of the boreal forest. The American Midland Naturalist 148(2): 282-288.
Hölker, F., C. Wolter, E. K. Perkin, and K. Tockner. 2010. Light pollution as a biodiversity threat. Trends in Ecology & Evolution 25(12): 681-682.
Holroyd, S. L., V. J. Craig, and P. Govindarajulu. 2016. Best Management Practices for Bats in British Columbia, Chapter 1: Introduction to the Bats of British Columbia. B.C. Ministry of Environment, Victoria, B.C.
Horacek, I., J. Zukal, T. Bartonika, and N. Martinkova. 2012. Ecology of geomycosis in central Europe. 5th Annual White-nose Syndrome Symposium, Madison,WI.
Horn, J. W., E. B. Arnett, and T. H. Kunz. 2008. Behavioral responses of bats to operating wind turbines. The Journal of Wildlife Management 72(1): 123-132.
Horváth, G., G. Kriska, P. Malik, and B. Robertson. 2009. Polarized light pollution: a new kind of ecological photopollution. Frontiers in Ecology and the Environment 7(6): 317-325.
Hoyt, J., K. Sun, K. Parise, G. Lu, K. Langwig, T. Jiang, S. Yang, W. Frick, A. Kilpatrick, and J. Foster. 2016. Widespread bat white-nose syndrome fungus, Northeastern China. Emerging Infectious Diseases 22(1).
Hoyt, J. R., T. L. Cheng, K. Langwig, M. M. Hee, W. F. Frick, and A. M. Kilpatrick. 2015a. Bacteria isolated from bats inhibit the growth of Pseudogymnoascus destructans, the causative agent of white-nose syndrome. PLoS One 10(4): e0121329.
Hoyt, J. R., K. E. Langwig, J. Okoniewski, W. F. Frick, W. B. Stone, and A. M. Kilpatrick. 2014. Long-term persistence of Pseudogymnoascus destructans, the causative agent of white-nose syndrome, in the absence of bats. EcoHealth: 1-4.
Hoyt, J. R., K. E. Langwig, J. Okoniewski, W. F. Frick, W. B. Stone, and A. M. Kilpatrick. 2015b. Long-Term Persistence of Pseudogymnoascus destructans, the Causative Agent of White-Nose Syndrome, in the Absence of Bats. EcoHealth 12(2): 330-333.
Humphries, M. M., D. W. Thomas, and J. R. Speakman. 2002. Climate-mediated energetic constraints on the distribution of hibernating mammals. Nature 418(6895): 313-316.
Huntington, T. G., A. D. Richardson, K. J. McGuire, and K. Hayhoe. 2009. Climate and hydrological changes in the northeastern United States: recent trends and implications for forested and aquatic ecosystems. Canadian Journal of Forest Research 39(2): 199-212.
Huynh, H. M. 2009. Another record of foliage roosting in the Little Brown Bat, Myotis lucifugus, in Canada. The Canadian Field-Naturalist 123(3): 265.
Johnson, L. N., B. A. McLeod, L. E. Burns, K. Arseneault, T. R. Frasier, and H. G. Broders. 2015. Population genetic structure within and among seasonal site types in the little brown bat (Myotis lucifugus) and the northern long-eared bat (M. septentrionalis). PLoS One 10(5): e0126309.
Jonasson, K. A. and C. K. Willis. 2011. Changes in body condition of hibernating bats support the thrifty female hypothesis and predict consequences for populations with white-nose syndrome. PLoS One 6(6): e21061.
Jones, G., D. S. Jacobs, T. H. Kunz, M. R. Willig, and P. A. Racey. 2009. Carpe noctem: the importance of bats as bioindicators. Endangered Species Research 8(1-2): 93-115.
Jones, G. and H. Rebelo. 2013. Responses of bats to climate change: learning from the past and predicting the future. Pages 457-478 In R. A. Adams and S. C. Pedersen (eds.). Bat Evolution, Ecology, and Conservation. Springer.
Jung, T. S., K. M. Blejwas, C. L. Lausen, J. M. Wilson, and L. E. Olson. 2014. Concluding remarks: what do we need to know about bats in northwestern North America? Northwestern Naturalist 95(3): 318-330.
Jung, T. S., B. G. Slough, D. W. Nagorsen, T. A. Dewey, and T. Powell. 2006. First records of the Northern Long-eared Bat, Myotis septentrionalis, in the Yukon Territory. The Canadian Field-Naturalist 120(1): 39-42.
Jung, T. S., I. D. Thompson, and R. D. Titman. 2004. Roost site selection by forest-dwelling male Myotis in central Ontario, Canada. Forest Ecology and Management 202(1): 325-335.
Jung, T. S., I. D. Thompson, R. D. Titman, and A. P. Applejohn. 1999. Habitat selection by forest bats in relation to mixed-wood stand types and structure in central Ontario. The Journal of Wildlife Management: 1306-1319.
Kalcounis-Rüppell, M. C., J. M. Psyllakis, and R. M. Brigham. 2005. Tree roost selection by bats: an empirical synthesis using meta-analysis. Wildlife Society Bulletin 33(3): 1123-1132.
Kannan, K., S. H. Yun, R. J. Rudd, and M. Behr. 2010. High concentrations of persistent organic pollutants including PCBs, DDT, PBDEs and PFOS in little brown bats with white-nose syndrome in New York, USA. Chemosphere 80(6): 613-618.
Karouna-Renier, N. K., C. White, C. R. Perkins, J. J. Schmerfeld, and D. Yates. 2014. Assessment of mitochondrial DNA damage in little brown bats (Myotis lucifugus) collected near a mercury-contaminated river. Ecotoxicology 23(8): 1419-1429.
Kilpatrick, M. 2013. Transmission of Geomyces destructans between bats and the environment. International Bat Research Conference San Jose, Costa Rica.
Kitzes, J. and A. Merenlender. 2014. Large roads reduce bat activity across multiple species. PLoS One 9(5): e96341.
Krusic, R. A., M. Yamasaki, C. D. Neefus, and P. J. Pekins. 1996. Bat habitat use in white mountain national forest. The Journal of Wildlife Management: 625-631.
Kuiper-Goodman, T., I. Falconer, and J. Fitzgerald. 1999. Human health aspects. Pages 113-153 In I. Chorus and J. Bartram (eds.). Toxic Cyanobacteria in Water, A Guide to Their Public Health Consequences, Monitoring and Management. Spon Press. New York, Ny.
Kunz, T. and M. Tuttle. 2009. White-nose syndrome science strategy meeting II., Austin, TX.
Kunz, T. H. 1973. Resource utilization: temporal and spatial components of bat activity in central Iowa. Journal of Mammalogy: 14-32.
Kunz, T. H. 1982. Roosting ecology in bats. Pages 1-56 In T. H. Kunz (ed.). Ecology of Bats. Plenum Press. New York, NY.
Kunz, T. H., E. B. Arnett, W. P. Erickson, A. R. Hoar, G. D. Johnson, R. P. Larkin, M. D. Strickland, R. W. Thresher, and M. D. Tuttle. 2007. Ecological impacts of wind energy development on bats: questions, research needs, and hypotheses. Frontiers in Ecology and the Environment 5(6): 315-324.
Kunz, T. H. and J. D. Reichard. 2010. Status review of the little brown myotis (Myotis lucifugus) and determination that immediate listing under the Endangered Species Act is scientifically and legally warranted. Boston University, Boston, Marsh.
Kunz, T. H. and D. S. Reynolds. 2003. Bat colonies in buildings. Pages 91-102 In T. J. O'Shea and M. A. Bogan, eds. Monitoring Trends in Bat Populations of the United States and Territories: Problems and Prospects. US Geological Survey, Springfield, VA.
Kunz, T. H., J. A. Wrazen, and C. D. Burnett. 1998. Changes in body mass and fat reserves in prehibernating little brown bats (Myotis lucifugus). Ecoscience: 8-17.
Kurta, A. 2014. The misuse of relative humidity in ecological studies of hibernating bats. Acta Chiropterologica 16(1): 249-254.
Kurta, A. and S. M. Smith. 2014. Hibernating bats and abandoned mines in the Upper Peninsula of Michigan. Northeastern Naturalist 21(4): 587-605.
Kyba, C. C., T. Ruhtz, J. Fischer, and F. Hölker. 2011. Cloud coverage acts as an amplifier for ecological light pollution in urban ecosystems. PLoS One 6(3): e17307-e17307.
Lacki, M. J., D. R. Cox, L. E. Dodd, and M. B. Dickinson. 2009. Response of Northern Bats (Myotis septentrionalis) to prescribed fires in Eastern Kentucky forests. Journal of Mammalogy 90(5): 1165-1175.
Lacoeuilhe, A., N. Machon, J.-F. Julien, A. Le Bocq, and C. Kerbiriou. 2014. The influence of low intensities of light pollution on bat communities in a semi-natural context. PLoS One 9(10): e103042.
Langwig, K., J. Voyles, M. Q. Wilber, W. F. Frick, K. A. Murray, B. M. Bolker, J. P. Collins, T. L. Cheng, M. C. Fisher, J. R. Hoyt, D. L. Lindner, H. I. McCallum, R. Puschendorf, E. B. Rosenblum, M. Toothman, C. K. R. Willis, C. J. Briggs, and A. M. Kilpatrick. 2015a. Context dependent conservation responses to emerging wildlife diseases. Frontiers in Ecology and the Environment 13: 195-202.
Langwig, K. E., W. F. Frick, J. T. Bried, A. C. Hicks, T. H. Kunz, and A. Marm Kilpatrick. 2012. Sociality, density‐dependence and microclimates determine the persistence of populations suffering from a novel fungal disease, white‐nose syndrome. Ecology Letters 15(9): 1050-1057.
Langwig, K. E., W. F. Frick, R. Reynolds, K. L. Parise, K. P. Drees, J. R. Hoyt, T. L. Cheng, T. H. Kunz, J. T. Foster, and A. M. Kilpatrick. 2015b. Host and pathogen ecology drive the seasonal dynamics of a fungal disease, white-nose syndrome. Proceedings of the Royal Society of London B: Biological Sciences 282(1799): 20142335.
Lausen, C. L., T. S. Jung, and J. M. Talerico. 2008. Range extension of the northern long-eared bat (Myotis septentrionalis) in the Yukon. Northwestern Naturalist 89(2): 115-117.
Le Roux, D. S. 2010. Monitoring long-tailed bat (Chalinolobus tuberculatus) activity and investigating the effect of aircraft noise on bat behaviour in a modified ecosystem. University of Waikato, Hamilton, New Zealand.
Leopardi, S., D. Blake, and S. J. Puechmaille. 2015. White-Nose Syndrome fungus introduced from Europe to North America. Current Biology 25(6): R217-R219.
Lesiński, G., A. Sikora, and A. Olszewski. 2011. Bat casualties on a road crossing a mosaic landscape. European Journal of Wildlife Research 57(2): 217-223.
Limpens, H. J. G. A., W. Helmer, A. Van Winden, and K. Mostert. 1989. Bats (Chiroptera) and linear landscape elements: a review of our present knowledge of the importance of linear landscape elements to bats. Lutra 32: 1-20.
Lindner, D. L., A. Gargas, J. M. Lorch, M. T. Banik, J. Glaeser, T. H. Kunz, and D. S. Blehert. 2011. DNA-based detection of the fungal pathogen Geomyces destructans in soils from bat hibernacula. Mycologia 103(2): 241-246.
Little, M. E., N. M. Burgess, H. Broders, and L. M. Campbell. 2015a. Distribution of mercury in archived fur from little brown bats across Atlantic Canada. Environmental Pollution 207: 52-28.
Little, M. E., N. M. Burgess, H. G. Broders, and L. M. Campbell. 2015b. Mercury in little brown bat (Myotis lucifugus) maternity colonies and its correlation with freshwater acidity in Nova Scotia, Canada. Environmental Science & Technology 49(4): 2059-2065.
Loeb, S. C. and J. M. O'Keefe. 2011. Bats and Gaps: The Role fo Early Successional Patches in the Roosting and Foraging Ecology of Bats. Pages 167-189 In C. Greenberg, B. Collins, and F. Thompson (eds.). Sustaining Young Forest Communities. Springer Netherlands.
Loeb, S. C., T. J. Rodhouse, L. E. Ellison, C. L. Lausen, J. D. Reichard, K. M. Irvine, T. E. Ingersoll, J. T. Coleman, W. E. Thogmartin, J. R. Sauer, C. M. Francis, M. L. Bayless, T. R. Stanley, and D. H. Johnson. 2015. A Plan for the North American Bat Monitoring Program (NABat). United States Department of Agriculture: Forest Service Research & Development, Asheville, NC.
Lorch, J. M., C. U. Meteyer, M. J. Behr, J. G. Boyles, P. M. Cryan, A. C. Hicks, A. E. Ballmann, J. T. Coleman, D. N. Redell, and D. M. Reeder. 2011. Experimental infection of bats with Geomyces destructans causes white-nose syndrome. Nature 480(7377): 376-378.
Lorch, J. M., L. K. Muller, R. E. Russell, M. O'Connor, D. L. Lindner, and D. S. Blehert. 2013. Distribution and environmental persistence of the causative agent of white-nose syndrome, Geomyces destructans, in bat hibernacula of the eastern United States. Applied and Environmental Microbiology 79(4): 1293-1301.
Loss, S. R., T. Will, and P. P. Marra. 2013. The impact of free-ranging domestic cats on wildlife of the United States. Nature 4: 1396.
Loyd, K. A. T., S. M. Hernandez, J. P. Carroll, K. J. Abernathy, and G. J. Marshall. 2013. Quantifying free-roaming domestic cat predation using animal-borne video cameras. Biological Conservation 160(0): 183-189.
Lučan, R. K., H. Bandouchova, T. Bartonička, J. Pikula, A. Zahradníková, J. Zukal, and N. Martínková. 2016. Ectoparasites may serve as vectors for the white-nose syndrome fungus. Parasites & Vectors 9(1): 1-5.
MacGregor, C. J., M. J. Pocock, R. Fox, and D. M. Evans. 2015. Pollination by nocturnal Lepidoptera, and the effects of light pollution: a review. Ecological Entomology 40(3): 187-198.
Maher, S. P., A. M. Kramer, J. T. Pulliam, M. A. Zokan, S. E. Bowden, H. D. Barton, K. Magori, and J. M. Drake. 2012. Spread of white-nose syndrome on a network regulated by geography and climate. Nature 3: 1306.
Main, A. R., J. V. Headley, K. M. Peru, N. L. Michel, A. J. Cessna, and C. A. Morrissey. 2014. Widespread use and frequent detection of neonicotinoid insecticides in wetlands of Canada's prairie pothole region. PLoS One 9(3): e92821.
Mainguy, J. and N. Desrosiers. 2011. Cave-dwelling bats in the province of Quebec: historical data about hibernacula population surveys. Ministère des Ressources naturelles et de la Faune, QC.
Marshall, B. 2016. Facts and Figures of the Canadian Mining Industry F&F 2016 (PDF).
Martínková, N., P. Bačkor, T. Bartonička, P. Blažková, J. Červený, and L. Falteisek. 2010. Increasing incidence of Geomyces destructans fungus in bats from the Czech Republic and Slovakia. PLoS One 5.
Masek, J. G., W. B. Cohen, D. Leckie, M. A. Wulder, R. Vargas, B. de Jong, S. Healey, B. Law, R. Birdsey, and R. Houghton. 2011. Recent rates of forest harvest and conversion in North America. Journal of Geophysical Research: Biogeosciences (2005–2012) 116(G4).
Maslo, B., M. Valent, J. F. Gumbs, and W. F. Frick. 2015. Conservation implications of ameliorating survival of little brown bats with white-nose syndrome. Ecological Applications 25(7): 1832-1840.
Mason, R., H. Tennekes, F. Sánchez-Bayo, and P. U. Jepsen. 2013. Immune suppression by neonicotinoid insecticides at the root of global wildlife declines. Journal of Environmental Immunology Toxicology 1(1): 3-12.
Master, L. L., D. Faber-Langendoen, R. Bittman, G. A. Hammerson, B. Heidel, L. Ramsay, K. Snow, A. Teucher, and A. Tomaino. 2012. NatureServe Conservation Status Assessments: Factors for Evaluating Species and Ecosystem Rank. NatureServe, Arlington, VA. [accessed: April 2016].
Mathews, F., N. Roche, T. Aughney, N. Jones, J. Day, J. Baker, and S. Langton. 2015. Barriers and benefits: implications of artificial night-lighting for the distribution of common bats in Britain and Ireland. Philosophical Transactions of the Royal Society of London B: Biological Sciences 370(1667): 20140124.
Matson, P. A., W. J. Parton, A. G. Power, and M. J. Swift. 1997. Agricultural intensification and ecosystem properties. Science 277(5325): 504-509.
Mattson, W. J. and R. A. Haack. 1987. The role of drought in outbreaks of plant-eating insects. BioScience: 110-118.
McAlpine, D., K. Vanderwolf, G. J. Forbes, and D. Malloch. 2011. Consumption of Bats (Myotis spp.) by Raccoons (Procyon lotor) During an Outbreak of White-Nose Syndrome in New Brunswick, Canada: Implications for Estimates of Bat Mortality. Vol. 125, No. 3, pp. 257-260..
McAlpine, D. F. 1983. Status and conservation of solution caves in New Brunswick. The New Brunswick Museum, St. John, NB.
McCracken, G. F. 2011. Cave conservation: special problems of bats. Pages 68-95 In J. Tyburec, J. Chenger, T. Snow, and C. Geiselman, eds. Bat Conservation International: Bat Conservation and Management Workshop. Bat Conservation International, Portal, AZ.
Medinas, D., J. T. Marques, and A. Mira. 2013. Assessing road effects on bats: the role of landscape, road features, and bat activity on road-kills. Ecological Research 28(2): 227-237.
Meteyer, C. U., E. L. Buckles, D. S. Blehert, A. C. Hicks, D. E. Green, V. Shearn-Bochsler, N. J. Thomas, A. Gargas, and M. J. Behr. 2009. Histopathologic criteria to confirm white-nose syndrome in bats. Journal of Veterinary Diagnostic Investigation 21(4): 411-414.
Mineau, P. and M. Whiteside. 2013. Pesticide acute toxicity is a better correlate of US grassland bird declines than agricultural intensification. PLoS One 8(2): e57457.
Moosman, P. R., H. H. Thomas, and J. P. Veilleux. 2012. Diet of the widespread insectivorous bats Eptesicus fuscus and Myotis lucifugus relative to climate and richness of bat communities. Journal of Mammalogy 93(2): 491-496.
Morrissey, C. A., P. Mineau, J. H. Devries, F. Sanchez-Bayo, M. Liess, M. C. Cavallaro, and K. Liber. 2015. Neonicotinoid contamination of global surface waters and associated risk to aquatic invertebrates: A review. Environment International 74: 291-303.
Mulec, J., E. Covington, and J. Walochnik. 2013. Is Bat Guano a Reservoir of Geomyces destructans? Open Journal of Veterinary Medicine 03(02): 161-167.
Murphy, M. 1987. Vandals destroy hibernating Indiana Bats. BATS Magazine 5(2): 5-8.
Nagorsen, D. and R. Brigham. 1993. Bats of British Columbia: Royal British Columbia museum handbook. University of British Columbia Press, Vancouver, BC.
NASBR. 2015. North American Society for Bat Research. Ypsilanti, MI. Available: . [accessed: 1 October 2015].
Natural Resources Canada. 2016. Biological control using Bacillus thuringiensis (B.t). Available:. [accessed: June 2016].
Natural Resources Canada. 2018. Forestery inventory. [accessed: October 2018].
Natureserve. 2015. NatureServe Explorer: An online encyclopedia of life [web application]. Version 7.0. Natureserve, Arlington, VA. U.S.A. [accessed: 20 April 2015].
Naughton, D. 2012. The Natural History of Canadian Mammals. University of Toronto Press. Toronto, ON. 784 pp.
Neilson, A. L. and M. B. Fenton. 1994. Responses of little brown myotis to exclusion and to bat houses. Wildlife Society Bulletin: 8-14.
NFD. 2014. National Forestry Database. Natural Resources Canada and Canadian Forest Service, Ottawa, ON. [accessed: 20 August 2014].
Norquay, K. J. O., F. Martinez-Nunez, J. E. Dubois, K. M. Monson, and C. K. R. Willis. 2013. Long-distance movements of little brown bats (Myotis lucifugus). Journal of Mammalogy 94(2): 506-515.
Northeast Bat Working Group. 2015. Northeast Bat Working Group. [accessed: 22 April 2015].
O'Regan, S. M., K. Magori, J. T. Pulliam, M. A. Zokan, R. R. B. Kaul, H. D. Barton, and J. M. Drake. 2014. Multi-scale model of epidemic fadeout: Will local extirpation events inhibit the spread of white-nose syndrome? Ecological Applications 25: 621-633.
O'Shea, T. J., P. M. Cryan, D. T. S. Hayman, R. K. Plowright, and D. G. Streicker. 2016. Multiple mortality events in bats: a global review. Mammal Review DOI: 10.1111/mam.12064.
O’Donoghue, A. J., G. M. Knudsen, C. Beekman, J. A. Perry, A. D. Johnson, J. L. DeRisi, C. S. Craik, and R. J. Bennett. 2015. Destructin-1 is a collagen-degrading endopeptidase secreted by Pseudogymnoascus destructans, the causative agent of white-nose syndrome. Proceedings of the National Academy of Sciences: 201507082.
Okoniewski, J., J. Haines, A. Hicks, K. Langwig, R. VonLinden, and C. Dobony. 2010. Detection of the conidia of Geomyces destructans in northeast hibernacula, at maternal colonies, and on gear – some findings based on microscopy and culture. Page 18 WNS Symposium, Pittsburg, Pennsylvania.
Olson, C. R. 2011. The roosting behaviour of Little Brown Bats (Myotis lucifugus) and Northern Long-eared Bats (Myotis septentrionalis) in the boreal forest of northern Alberta. University of Calgary, Calgary, AB.
Olson, C. R. and R. M. Barclay. 2013. Concurrent changes in group size and roost use by reproductive female little brown bats (Myotis lucifugus). Canadian Journal of Zoology 91(3): 149-155.
Olson, C. R., D. P. Hobson, and M. J. Pybus. 2011. Changes in population size of bats at a hibernaculum in Alberta, Canada, in relation to cave disturbance and access restrictions. Northwestern Naturalist 92(3): 224-230.
OMNRF. 2015. Ontario: Species at Risk. [accessed: 3 May 2015].
Ontario Ministry of Finance. 2016. Ontario Population Projections Update, 2015-2041. Page 111 pp. In O. M. o. Finance, ed.
Ontario Ministry of Natural Resources. 2010. Forest Management Guide for Conserving Biodiversity at the Stand and Site Scales. Ontario Ministry of Natural Resources, Ontario.
Ontario Ministry of Natural Resources and Forestry. 2015. Ontario's White-nose Syndrome Response Plan. Wildlife Section, Species Conservation Policy Branch, Ontario.
Owen, S. F., M. A. Menzel, W. M. Ford, B. R. Chapman, K. V. Miller, J. W. Edwards, and P. B. Wood. 2003. Home-range size and habitat used by the northern myotis (Myotis septentrionalis). The American Midland Naturalist 150(2): 352-359.
Park, A. C. and H. G. Broders. 2012. Distribution and roost selection of bats on Newfoundland. Northeastern Naturalist 19(2): 165-176.
Patriquin, K. J., F. Palstra, M. L. Leonard, and H. G. Broders. 2013. Female northern myotis (Myotis septentrionalis) that roost together are related. Behavioral Ecology 24(4): 949-954.
Pauli, B., P. Zollner, G. Haulton, and G. Shao. 2015. The simulated effects of timber harvest on suitable habitat for Indiana and northern long-eared bats.
Perkin, E. K., F. Hölker, and K. Tockner. 2014. The effects of artificial lighting on adult aquatic and terrestrial insects. Freshwater Biology 59(2): 368-377.
Perry, R. and R. Thill. 2007. Tree roosting by male and female eastern pipistrelles in a forested landscape. Journal of Mammalogy 88: 974-981.
Petrick, S. 2015. Tyendinaga Cavern and Caves opens for summer. Belleville News, Belleville, Ontario.
Pikula, J., H. Bandouchova, L. Novotný, C. U. Meteyer, J. Zukal, N. R. Irwin, J. Zima, and N. Martínková. 2012. Histopathology confirms white-nose syndrome in bats in Europe. Journal of Wildlife Diseases 48(1): 207-211.
Poissant, J. 2009. Roosting and social ecology of the tri-colored bat, Perimyotis subflavus, in Nova Scotia. St. Mary's University, Halifax, NS.
Poissant, J. A., H. G. Broders, and G. M. Quinn. 2010. Use of lichen as a roosting substrate by Perimyotis subflavus, the tricolored bat, in Nova Scotia. Ecoscience 17(4): 372-378.
Porvari, P., M. Verta, J. Munthe, and M. Haapanen. 2003. Forestry practices increase mercury and methyl mercury output from boreal forest catchments. Environmental Science & Technology 37(11): 2389-2393.
Powers, L., E. Pritchard, K. Lau, and B. Francis. 2012. Does Geomyces destructans infection reduce female fertility in Myotis lucifugus? . North America Symposium on Bat Research, Puerto Rico.
Puechmaille, S. J., G. Wibbelt, V. Korn, H. Fuller, F. Forget, K. Muhldorfer, A. Kurth, W. Bogdanowicz, C. Borel, T. Bosch, T. Cherezy, M. Drebet, T. Gorfol, A. J. Haarsma, F. Herhaus, G. Hallart, M. Hammer, C. Jungmann, Y. Le Bris, L. Lutsar, M. Masing, B. Mulkens, K. Passior, M. Starrach, A. Wojtaszewski, U. Zophel, and E. C. Teeling. 2011. Pan-European distribution of white-nose syndrome fungus (Geomyces destructans) not associated with mass mortality. PLoS One 6(4): e19167.
Pybus, M., D. Hobson, and D. Onderka. 1986. Mass mortality of bats due to probable blue-green algal toxicity. Journal of Wildlife Diseases 22(3): 449-450.
Quinn, G. M. and H. G. Broders. 2007. Roosting and foraging ecology of eastern pipistrelle (Perimyotis subflavus) bats in SW Nova Scotia. Nova Scotia Habitat Conservation Fund c/o NS Department of Natural Resources, Halifax, NS.
Raesly, R. L. and J. E. Gates. 1987. Winter habitat selection by north temperate cave bats. American Midland Naturalist: 15-31.
Randall, J. and H. G. Broders. 2014. Identification and characterization of swarming sites used by bats in Nova Scotia, Canada. Acta Chiropterologica 16(1): 109-116.
Randall, L. A., T. S. Jung, and R. M. Barclay. 2014. Roost-site selection and movements of Little Brown Myotis (Myotis lucifugus) in southwestern Yukon. Northwestern Naturalist 95(3): 312-317.
Ratcliffe, J. M. and J. W. Dawson. 2003. Behavioural flexibility: the little brown bat, Myotis lucifugus, and the northern long-eared bat, M. septentrionalis, both glean and hawk prey. Animal Behaviour 66(5): 847-856.
Raudabaugh, D. B. and A. N. Miller. 2013. Nutritional capability of and substrate suitability for Pseudogymnoascus destructans, the causal agent of bat white-nose syndrome. PLoS One 8(10): e78300.
Raudabaugh, D. B. and A. N. Miller. 2015. Effects of trans, trans-farnesol on Pseudogymnoascus destructans and several closely related species. Mycopathologia.
Rebelo, H. and A. Rainho. 2009. Bat conservation and large dams: spatial changes in habitat use caused by Europe's largest reservoir. Endangered Species Research 8: 61-68.
Reeder, D. and G. Turner. 2008. Working together to combat white-nose syndrome: a report of a meeting on 9–11 June 2008. Bat Research News 49(7).
Reeder, D. M., C. L. Frank, G. G. Turner, C. U. Meteyer, A. Kurta, E. R. Britzke, M. E. Vodzak, S. R. Darling, C. W. Stihler, and A. C. Hicks. 2012. Frequent arousal from hibernation linked to severity of infection and mortality in bats with white-nose syndrome. PLoS One 7(6): e38920.
Reichard, J. D., N. W. Fuller, A. B. Bennett, S. R. Darling, M. S. Moore, K. E. Langwig, E. D. Preston, S. v. Oettingen, C. S. Richardson, and D. Scott Reynolds. 2014. Interannual survival of Myotis lucifugus (Chiroptera: Vespertilionidae) near the epicenter of white-nose syndrome. Northeastern Naturalist 21(4): N56-N59.
Reichard, J. D. and T. H. Kunz. 2009. White-nose syndrome inflicts lasting injuries to the wings of little brown myotis (Myotis lucifugus). Acta Chiropterologica 11(2): 457-464.
Reimer, J. and L. Kaupas. 2013. South Slave region bat research summary 2013: University of Calgary. Environment and Natural Resources, Government of Northwest Territiories, Fort Smith, NT.
Reimer, J. P. 2013. Nocturnality under the mignight sun: behavioural adaptations of the little brown bat (Myotis lucifugus) at 60° N latitude, Calgary, AB.
Ren, P., K. H. Haman, L. A. Last, S. S. Rajkumar, M. K. Keel, and V. Chaturvedi. 2012. Clonal spread of Geomyces destructans among bats, Midwestern and Southern United States. Emergent Infectious Disease 18(5): 883-885.
Reynolds, D. S. 2006. Monitoring the potential impact of a wind development site on bats in the northeast. Journal of Wildlife Management 70(5): 1219-1227.
Reynolds, H. T. and H. A. Barton. 2014. Comparison of the white-nose syndrome agent Pseudogymnoascus destructans to cave-dwelling relatives suggests reduced saprotrophic enzyme activity. PLoS One 9(1): e86437.
Reynolds, H. T., T. Ingersoll, and H. A. Barton. 2015. Modeling the environmental growth of Pseudogymnoascus destructans and its impact on the white-nose syndrome epidemic. J Wildl Dis 51(2): 318-331.
Rodrigue, J. L., T. M. Schuler, and M. A. Menzel. 2001. Observations of bat activity during prescribed burning in West Virginia. Bat Research News 42: 48-49.
Rollins, K. E., D. K. Meyerholz, G. D. Johnson, A. P. Capparella, and S. S. Loew. 2012. A forensic investigation into the etiology of bat mortality at a wind farm: barotrauma or traumatic injury? Veterinary Pathology Online 49(2): 362-371.
Russell, A., M. Vonhof, and E. Claire. 2012. Phylogeographic analyses reveal cryptic subdivisions and unexpected connections among Myotis lucifugus populations. North America Symposium on Bat Research, Puerto Rico.
Russell, A. L., C. M. Butchkoski, L. Saidak, and G. F. McCracken. 2009. Road-killed bats, highway design, and the commuting ecology of bats. Endangered Species Research 8: 49-60.
Rydell, J., L. Bach, P. Bach, L. G. Diaz, J. Furmankiewicz, N. Hagner-Wahlsten, E.-M. Kyheröinen, T. Lilley, M. Masing, and M. M. Meyer. 2014. Phenology of migratory bat activity across the Baltic Sea and the south-eastern North Sea. Acta Chiropterologica 16(1): 139-147.
Sandel, J. K., G. R. Benatar, K. M. Burke, C. W. Walker, J. Lacher, Thomas E, and R. L. Honeycutt. 2001. Use and selection of winter hibernacula by the eastern pipistrelle (Pipistrellus subflavus) in Texas. Journal of Mammalogy 82(1): 173-178.
Sasse, D. and P. Perkins. 1996. Summer roosting ecology of northern long-eared bats (Myotis septentrionalis) in the White Mountain National Forest. Pages 91-101 In Bats and Forests Symposium. British Columbia Ministry of Forests Victoria, BC.
Scott, F. W. and A. J. Hebda. 2004. Annotated list of the mammals of Nova Scotia. Proceedings of the Nova Scotian Institute of Science (NSIS) 42(2).
Segers, J. and H. Broders. 2014. Interspecific effects of forest fragmentation on bats. Canadian Journal of Zoology 92(8): 665-673.
Segers, J. L. and H. G. Broders. 2015. Carbon (δ 13 C) and nitrogen (δ 15 N) stable isotope signatures in bat fur indicate swarming sites have catchment areas for bats from different summering areas. PLoS One 10(4): e0125755.
Shelley, V., S. Kaiser, E. Shelley, T. Williams, M. Kramer, K. Haman, K. Keel, and H. Barton. 2013. Evaluation of Strategies for the Decontamination of Equipment for Geomyces destructans, the Causative Agent of the White-Nose Syndrome (WNS). Journal of Cave and Karst Studies: 1-10.
Sherwin, H. A., W. I. Montgomery, and M. G. Lundy. 2012. The impact and implications of climate change for bats. Mammal Review 43(3): 171-182.
Silvis, A., W. M. Ford, and E. R. Britzke. 2015a. Day-roost tree selection by northern long-eared bats—What do non-roost tree comparisons and one year of data really tell us? Global Ecology and Conservation 3(0): 756-763.
Silvis, A., W. M. Ford, and E. R. Britzke. 2015b. Effects of hierarchical roost removal on Northern Long-Eared Bat (Myotis septentrionalis) maternity colonies. PLoS One 10(1).
Sivonen, K. and G. Jones. 1999. Cyanobacterial toxins. Pages 41-111 In I. Chorus and J. Bartram (eds.). Toxic Cyanobacteria in Water, a Guide to Their Public Health Consequences, Monitoring and Management. Spon Press. New York, NY.
Slough, B. G. 2009. Behavioral thermoregulation by a maternity colony of little brown bats in the Yukon. Northwestern Naturalist: 47-51.
Slough, B. G. and T. S. Jung. 2007. Diversity and distribution of the terrestrial mammals of the Yukon Territory: A review. The Canadian Field-Naturalist 121(2): 119-127.
Slough, B. G. and T. S. Jung. 2008. Observations on the natural history of bats in the Yukon. Northern Review(29): 127-150.
Smallwood, K. S. 2013. Comparing bird and bat fatality‐rate estimates among North American wind‐energy projects. Wildlife Society Bulletin 37(1): 19-33.
Smith, J. L., G. L. Boyer, E. Mills, and K. L. Schiulz. 2008. Toxicity of microcystin-LR, a cyanobacterial toxin, to multiple life stages of the burroeing mayfly, Hexagenia, and possible implications for recruitment. Environmental Toxicoloogy 23(4): 499-506.
Spanjer, G. R. and M. B. Fenton. 2005. Behavioral responses of bats to gates at caves and mines. Wildlife Society Bulletin 33(3): 1101-1112.
Sparks, T. C. 2013. Insecticide discovery: an evaluation and analysis. Pesticide biochemistry and physiology 107(1): 8-17.
Speakman, J., P. Webb, and P. Racey. 1991. Effects of disturbance on the energy expenditure of hibernating bats. Journal of Applied Ecology: 1087-1104.
SSM. 2015. Speleological Society of Manitoba. Speleological Society of Manitoba. [accessed: 16 July 2015].
Statistics Canada. 2018. Human Activity and the Environment. [accessed: October 2018].
Stone, E. L., G. Jones, and S. Harris. 2009. Street lighting disturbs commuting bats. Current Biology 19(13): 1123-1127.
Swystun, M. B., J. M. Psyllakis, and R. M. Brigham. 2001. The influence of residual tree patch isolation on habitat use by bats in central British Columbia. Acta Chiropterologica 3(2): 197-201.
Syme, D. M., M. B. Fenton, and J. Zigouris. 2001. Roosts and food supplies ameliorate the impact of a bad summer on reproduction by the bat, Myotis lucifugus LeConte (Chiroptera: Vespertilionidae). Ecoscience: 18-25.
Talerico, J. M. 2008. The behaviour, diet and morphology of the little brown bat (Myotis lucifugus) near the northern extent of its range in Yukon Canada. University of Calgary, Calgary, AB.
Thomas, D. W. 1995. Hibernating bats are sensitive to nontactile human disturbance. Journal of Mammalogy 76(3): 940-946.
Thomas, D. W., M. Dorais, and J.-M. Bergeron. 1990. Winter energy budgets and cost of arousals for hibernating little brown bats, Myotis lucifugus. Journal of Mammalogy: 475-479.
Thomas, D. W., M. B. Fenton, and R. Barclay. 1979. Social behaviour of the little brown bat, Myotis lucifugus Behavioral Ecology and Sociobiology 6(2): 129-136.
Thomas, H. H., P. R. Moosman, J. P. Veilleux, and J. Holt. 2012. Foods of bats (Family Vespertilionidae) at five locations in New Hampshire and Massachusetts. The Canadian Field-Naturalist 126(2): 117-124.
Thorne, T. J. 2015. The role of islands in the migration of bats across Lake Erie and Lake Ontario: Lasiurus borealis, Lasiurus cinereus and Perimyotis subflavus. The University of Western Ontario, London, ON.
Trombulak, S. C., P. E. Higuera, and M. DesMeules. 2001. Population trends of wintering bats in Vermont. Northeastern Naturalist 8(1): 51-62.
Turner, G. G., D. Reeder, and J. T. Coleman. 2011. A five-year assessment of mortality and geographic spread of white-nose syndrome in North American bats, with a look at the future. Update of white-nose syndrome in bats. Bat Research News: 13.
Turner, R. W. 1974. Mammals of the Black Hills of South Dakota and Wyoming. University of Kansas. Lawrence, KS. pp.
U.S. Fish and Wildlife Service. 2007. Indiana Bat (Myotis sodalis) draft recovery plan: first revision. U.S. Fish and Wildlife Service, Fort Snelling, MN.
U.S. Fish and Wildlife Service. 2012. North American bat death toll exceeds 5.5 million from white-nose syndrome. U.S. Fish and Wildlife Service, Arlington, VA.
U.S. Fish and Wildlife Service. 2016. White-nose Syndrome. Hadley, Marsh. Available: www.whitenosesyndrome.org. [accessed: 6 April 2016].
U.S. Fish and Wildlife Service. 2018. White-nose Syndrome. Hadley, Marsh. Available: www.whitenosesyndrome.org. [accessed: 29 May 2018].
U.S. Geological Survey. 2000. Mercury in the environment, fact sheet 146-00. Available: http://www.usgs.gov/themes/factsheet/146-00/ [accessed: August 2014].
van Zyll de Jong, C. G. 1985. Handbook of Canadian Mammals: Bats. National Museum of Natural Sciences. Ottawa, ON. 212 pp.
Vanderwolf, K. J., D. F. McAlpine, G. J. Forbes, and D. Malloch. 2012. Bat populations and cave microclimate prior to and at the outbreak of white-nose syndrome in New Brunswick. The Canadian Field-Naturalist 126(2): 125-134.
Veilleux, J. P. and S. L. Veilleux. 2004. Intra-annual and interannual fidelity to summer roost areas by female eastern pipistrelles, Pipistrellus subflavus. The American Midland Naturalist 152(1): 196-200.
Veilleux, J. P., J. O. Whitaker Jr, and S. L. Veilleux. 2003. Tree-roosting ecology of reproductive female eastern pipistrelles, Pipistrellus subflavus, in Indiana. Journal of Mammalogy 84(3): 1068-1075.
Verant, M. L., M. U. Carol, J. R. Speakman, P. M. Cryan, J. M. Lorch, and D. S. Blehert. 2014. White-nose syndrome initiates a cascade of physiologic disturbances in the hibernating bat host. BMC Physiology 14(1): 10.
Verboom, B. and K. Spoelstra. 1999. Effects of food abundance and wind on the use of tree lines by an insectivorous bat, Pipistrellus pipistrellus. Canadian Journal of Zoology 77: 1393-1401.
Vuori, K.-M., O. Siren, and H. Luotonen. 2003. Metal contamination of streams in relation to catchment silvicultural practices: a comparative study in Finnish and Russian headwaters. Boreal Environment Research 8(1): 61-70.
Warnecke, L., J. M. Turner, T. K. Bollinger, J. M. Lorch, V. Misra, P. M. Cryan, G. Wibbelt, D. S. Blehert, and C. K. Willis. 2012. Inoculation of bats with European Geomyces destructans supports the novel pathogen hypothesis for the origin of white-nose syndrome. Proceedings of the National Academy of Sciences 109(18): 6999-7003.
Warnecke, L., J. M. Turner, T. K. Bollinger, V. Misra, P. M. Cryan, D. S. Blehert, G. Wibbelt, and C. K. Willis. 2013. Pathophysiology of white-nose syndrome in bats: a mechanistic model linking wing damage to mortality. Biology Letters 9(4): 20130177.
Webb, P., J. Speakman, and P. Racey. 1995. Evaporative water loss in two sympatric species of vespertilionid bat, Plecotus auritus and Myotis daubentoni: relation to foraging mode and implications for roost site selection. Journal of Zoology 235(2): 269-278.
Western Bat Working Group. 2015. Western Bat Working Group. [accessed: 22 April 2015].
Whitaker, J. O. and L. J. Rissler. 1993. Do bats feed in winter? American Midland Naturalist: 200-203.
Wibbelt, G., A. Kurth, D. Hellmann, M. Weishaar, A. Barlow, M. Veith, J. Prüger, T. Görföl, L. Grosche, and F. Bontadina. 2010. White-nose syndrome fungus (Geomyces destructans) in bats, Europe. Emerging Infectious Diseases 16(8): 1237.
Wickramasinghe, L. P., S. Harris, G. Jones, and N. Vaughan. 2003. Bat activity and species richness on organic and conventional farms: impact of agricultural intensification. Journal of Applied Ecology 40(6): 984-993.
Wiener, J. G., D. P. Krabbenhoft, G. H. Heinz, and A. M. Scheuhammer. 2003. Ecotoxicology of mercury. Pages 409-463 In D. J. Hoffman, B. A. Rattner, G. A. Burton, Jr., and J. Cairns, Jr. (eds.). Handbook of Ecotoxicology, 2nd Edition. CRC Press. Boca Raton, FL.
Wilder, A. P. 2014. Spatial, ecological and genetic correlates of the geographic expansion of an infectious disease, white-nose syndrome in bats. University of California, Santa Cruz, CA.
Willis, C. K. and R. M. Brigham. 2007. Social thermoregulation exerts more influence than microclimate on forest roost preferences by a cavity-dwelling bat. Behavioral Ecology and Sociobiology 62(1): 97-108.
Wilson, J. M. and R. M. Barclay. 2006. Consumption of caterpillars by bats during an outbreak of western spruce budworm. The American Midland Naturalist 155(1): 244-249.
Wilson, J. M., J. P. Reimer, D. Allaire, and C. L. Lausen. 2014. Diversity and distribution of bats in the Northwest Territories. Northwestern Naturalist 95(3): 197-218.
Woller-Skar, M. M., D. N. Jones, M. R. Luttenton, and A. L. Russell. 2015. Mycrocystin detected in little brown bats (Myotis lucifugus). The American Midland Naturalist 174(2): 331-334.
Xing, Z., L. Chow, H. Rees, F. Meng, S. Li, B. Ernst, G. Benoy, T. Zha, and L. M. Hewitt. 2013. Influences of sampling methodologies on pesticide-residue detection in stream water. Archives of Environmental Contamination and Toxicology 64(2): 208-218.
Yates, D. E., E. M. Adams, S. E. Angelo, D. C. Evers, J. Schmerfeld, M. S. Moore, T. H. Kunz, T. Divoll, S. T. Edmonds, and C. Perkins. 2014. Mercury in bats from the northeastern United States. Ecotoxicology 23(1): 45-55.
Young, J. E., G. A. Sánchez-Azofeifa, S. J. Hannon, and R. Chapman. 2006. Trends in land cover change and isolation of protected areas at the interface of the southern boreal mixedwood and aspen parkland in Alberta, Canada. Forest Ecology and Management 230(1–3): 151-161.
Zimmerling, R. and C. Francis. 2016. Bat mortality due to wind turbines in Canada. Journal of Wildlife Management 80(8): 1360-1369.
Zimmerman, G. S. and W. E. Glanz. 2000. Habitat use by bats in eastern Maine. The Journal of Wildlife Management 64(4): 1032-1040.
Zukal, J., H. Bandouchova, J. Brichta, A. Cmokova, K. S. Jaron, M. Kolarik, V. Kovacova, A. Kubatova, A. Novakova, O. Orlov, J. Pikula, P. Presetnik, J. Suba, A. Zahradnikova, Jr., and N. Martinkova. 2016. White-nose syndrome without borders: Pseudogymnoascus destructans infection tolerated in Europe and Palearctic Asia but not in North America. Sci Rep 6: 19829.
Personal Communications
Blejwas, K. 2015. Regional Wildlife Biologist, Alaska Department of Fish and Game, Juneau, AK.
Brigham, M. 2015. Professor, University of Regina, Regina, SkON.
Broders, H. 2015. Associate Professor, St. Mary’s University, Halifax, NS.
Davis, M. 2016. Island Karst Research, Tahsis, BC.
Govindarajulu, P. 2015. Amphibian, Reptile, and Small Mammal Specialist, British Columbia Ministry of Environment, Victoria, BC.
Hobson, D. 2015. Senior Wildlife Biologist, Alberta Environment and Parks, Edson, AB
Horne, G. 2015. Resource Management Officer, Jasper National Park, Jasper, AB.
Jung, T. 2015. Senior Wildlife Biologist, Whitehorse, YK.
Lausen, C. 2015. Research Biologist, Bat Specialist, Wildlife Conservation Society Canada, Kaslo, BC.
Morningstar, D. 2015. Terrestrial Ecologist, Golder Associates Ltd, Cambridge, ON.
Pardy-Moores, S. 2015. Senior Manager – Endangered Species and Biodiversity, Department of Environment and Conservation, Corner Brook, NL.
Appendix A: Effects on the environment and other species
A strategic environmental assessment (SEA) is conducted on all SARA recovery planning documents, in accordance with the Cabinet Directive on the Environmental Assessment of Policy, Plan and Program Proposals. The purpose of a SEA is to incorporate environmental considerations into the development of public policies, plans, and program proposals to support environmentally sound decision-making and to evaluate whether the outcomes of a recovery planning document could affect any component of the environment or any of the Federal Sustainable Development Strategy’s (FSDS) goals and targets.
Recovery planning is intended to benefit species at risk and biodiversity in general. However, it is recognized that strategies may also inadvertently lead to environmental effects beyond the intended benefits. The planning process based on national guidelines directly incorporates consideration of all environmental effects, with a particular focus on possible impacts upon non-target species or habitats. The results of the SEA are incorporated directly into the strategy itself, but are also summarized below in this statement.
WNS affects other bat species not considered in this recovery strategy (e.g., Eastern Small-footed Myotis – Myotis leibii) (U.S. Fish and Wildlife Service 2018). Therefore, any approaches that mitigate the impact or spread of WNS will most likely also benefit these species. In contrast, it is possible that other species of bats with populations not heavily impacted by WNS (e.g., Big Brown Bat – Eptesicus fuscus) may be benefiting from the declining populations of Little Brown Myotis, Northern Myotis, and Tri-colored Bat by filling the niche recently vacated (Francl et al. 2012). It is unknown how recovery of the three at-risk species will affect these other bat species that have recently increased.
Many potential threats identified in this recovery strategy have also been identified as threats for other species at risk. Approaches that help to minimize these threats may also benefit other species. For example, Rusty Blackbird (Euphagus carolinus) is thought to be susceptible to mercury contamination in Eastern Canada (Edmonds et al. 2010), and feral and free-roaming cats have been identified as a potential threat to numerous bird species (Calvert et al. 2013), including other species at risk, such as Common Nighthawk (Chordeiles minor) (Environment Canada 2016). Conservation of forests surrounding hibernacula and roosts may positively affect other species (at local scales) that are also threatened by forest removal (e.g., Canada Warbler – Cardellina canadensis and Woodland Caribou – Rangifer tarandus caribou) (Environment Canada 2012, 2015).
Bat populations consume substantial quantities of insects each night and therefore control the local populations of insects. Initiatives that result in the recovery of bat populations may cause local declines in insect populations (some of which may have already exhibited drastic declines) (Dirzo et al. 2014). In contrast, strategies that investigate the declining insect prey, or research, mitigate, or educate the public about mutual potential threats may aid in the recovery of possible depleted insect populations.
The possibility that the present recovery strategy inadvertently generates negative effects on the environment and on other species was considered. The majority of recommended actions are non-intrusive in nature, including surveys, research, and outreach. It is unlikely that the present recovery strategy will produce significant negative effects.
Appendix B: Additional research needs related to known and suspected threats
The following list is not exhaustive (nor in order of priority), and illustrates some of the research required to understand the threats (other than WNS) to Little Brown Myotis, Northern Myotis, and Tri-colored Bat and their habitats.
Energy production and mining (The median threat rate impact is either high or medium)
- Further investigate the location and characteristics of wind turbines in Canada that may significantly affect bat populations
- Further investigate the timing and population-level effects of wind turbines and continue to develop techniques to reduce mortality
- Continue to monitor frequency of wind turbine collisions in Canada, and develop a consistent range-wide monitoring program for wind energy facilities
Pollution (The median threat rate impact is medium)
- Further investigate the bats’ exposure to mercury and other pollutants across their ranges
- Determine the potential effects of mercury on biology, survival, and behavior
- Determine the effects of neonicotinoids and other widely-used pesticides on bats
- Determine the effects of techniques to reduce the spread of Spruce Budworm on bats
- Determine the effects of light pollution on bat behavior, foraging efficiency, and their prey
Human intrusion and disturbance (The median threat rate impact is low)
- Determine the effects of different levels and types of noise on behavior and biology of bats throughout their life-cycles
- Determine the effects of research activities on bat stress and survival
- Investigate the effects and characteristics of vehicle-bat collisions in Canada
- Investigate the effects of bat collisions related to non-traditional / recreational vehicles and devices (e.g., boats, unmanned aerial vehicles, and fishing lines)
Biological resource use (The medium threat rate impact is low)
- Determine the effects of common forestry operations (e.g., silviculture, and selective cutting) on roost tree availability, behavior, biology, and movement patterns across the range of the species
- Determine the amount (and characteristics) of forest removal, harvesting, and silviculture that can be completed while maintaining enough suitable habitat for bat populations across the range of the species
- Continue to investigate the effects of forest fragmentation from various sources (e.g., agriculture and road development)
- Investigate the significance of habitat loss due to insect outbreaks (e.g., Mountain Pine Beetle)
- Further investigate the effects of exclusion of maternity colonies from anthropogenic structures
- Further investigate the use of bat boxes to mitigate loss of anthropogenic or natural roosting structures
Natural system modifications (The median threat rate impact is low)
- Investigate the potential impact of insect outbreaks and forest fires on these species, their prey, and their habitat
Invasive & Other problematic species & genes (The median threat rate impact is very high because of WNS)
- Determine human-related predation risk in urban and rural areas (e.g., by cats)
Climate change (The median threat rate impact is unknown)
- Determine the impacts of climate change on these species, their prey, and their habitat
Footnotes
- footnote[1] Back to paragraph These federally protected areas are: a national park of Canada named and described in Schedule 1 to the Canada National Parks Act, The Rouge National Park established by the Rouge National Urban Park Act, a marine protected area under the Oceans Act, a migratory bird sanctuary under the Migratory Birds Convention Act, 1994 or a national wildlife area under the Canada Wildlife Act see ss. 58(2) of SARA.
- footnote[2] Back to paragraph Tragus is a prominence on the inner side of the external ear.
- footnote[3] Back to paragraph Extralimital observations refer to observations that occur outside the defined range of the species.
- footnote[4] Back to paragraph Functionally extirpated populations are those of which there are so few remaining numbers that there are not enough individuals or habitat in suitable condition to support a fully functional population (NOAA 2015).
- footnote[6] Back to paragraph Philopatry is the tendency to return to the home area.
- footnote[7] Back to paragraph Gleaning is the act of taking prey off a substrate rather than in the air or water.
- footnote[8] Back to paragraph Additive to existing natural sources of mortality. For example, mortality from collisions with wind turbines and vehicles; exclusion practices that result in mortality; predation by cats; and WNS.
- footnote[9] Back to paragraph The overall threat impact was calculated following Master et al. (2012) using the number of Level 1 Threats assigned to each species where timing = High. For Little Brown Myotis, this included 1 Very High, 1 High, 1 Medium, 6 Low (Table 2). For Northern Myotis, this included 1 Very High, 2 Medium, 5 Low, 1 Negligible (Table 3). For Tri-colored Bat, this included 1 Very High, 2 Medium, 6 Low (Table 4).The overall threat impact considers the cumulative impacts of multiple threats.
- footnote[10] Back to paragraph Confidence Interval (C. I.) - a range of values presented with a specified probability that the actual value of the parameter lies within the range. In this case, there is a 95% probability that the actual number of bats killed annually (as of December 2013) by wind turbines in Canada is between 32,100 and 62,700.
- footnote[11] Back to paragraph Histoplasmosis is an infection caused by a fungus that can be found in bat feces.
- footnote[12] Back to paragraph Fungi that can cause infections of the skin, hair, and nails due to their ability to utilize keratin.
- footnote[13] Back to paragraph Flutter-detectors use high duty cycles of echolocation to locate fluttering insect prey. Species that use high duty cycles are able to separate pulse and echo by frequency; species that use low duty cycles separate pulse and echo by time.
- footnote[14] Back to paragraph Aerial hawkers catch flying insects while on the wing (i.e., flying). In bats, echolocation is used to locate prey.
- footnote[15] Back to paragraph Disease surveillance. Courtesy of United States Geological Survey (USGS) and Canadian Wildlife Health Cooperative (CWHC).
- footnote[16] Back to paragraph The invasion front is where P. destructans has just arrived but prevalence is low or where arrival is imminent (Langwig et al. 2015a).
- footnote[17] Back to paragraph The objective will be measured against each 6-year cumulative interval (i.e., at 6 years [year 1 to 6 combined], at 12 years [year 1 to 12 combined] and at 18 years [year 1 to18 combined]).
- footnote[18] Back to paragraph The term self-sustaining refers to a population that does not require human intervention for long-term persistence.
- footnote[19] Back to paragraph The term resilient refers to a population that is of sufficient size to recover from periodic disturbance and avoid genetic collapse.
- footnote[20] Back to paragraph The term redundant refers to a population with sufficient subpopulations available to withstand catastrophic events and facilitate rescue if necessary.
- footnote[21] Back to paragraph The term representative refers to a population with presence across the diversity of ecosystems it inhabits and of the species roles in ecosystem processes.
- footnote[22] Back to paragraph The objective will be measured against each 5-year cumulative interval (i.e., at 5 years [year 1 to 5 combined] and at 10 years [year 1 to10 combined]).